3.6 Amino Acids Per One Alpha Helix Turn
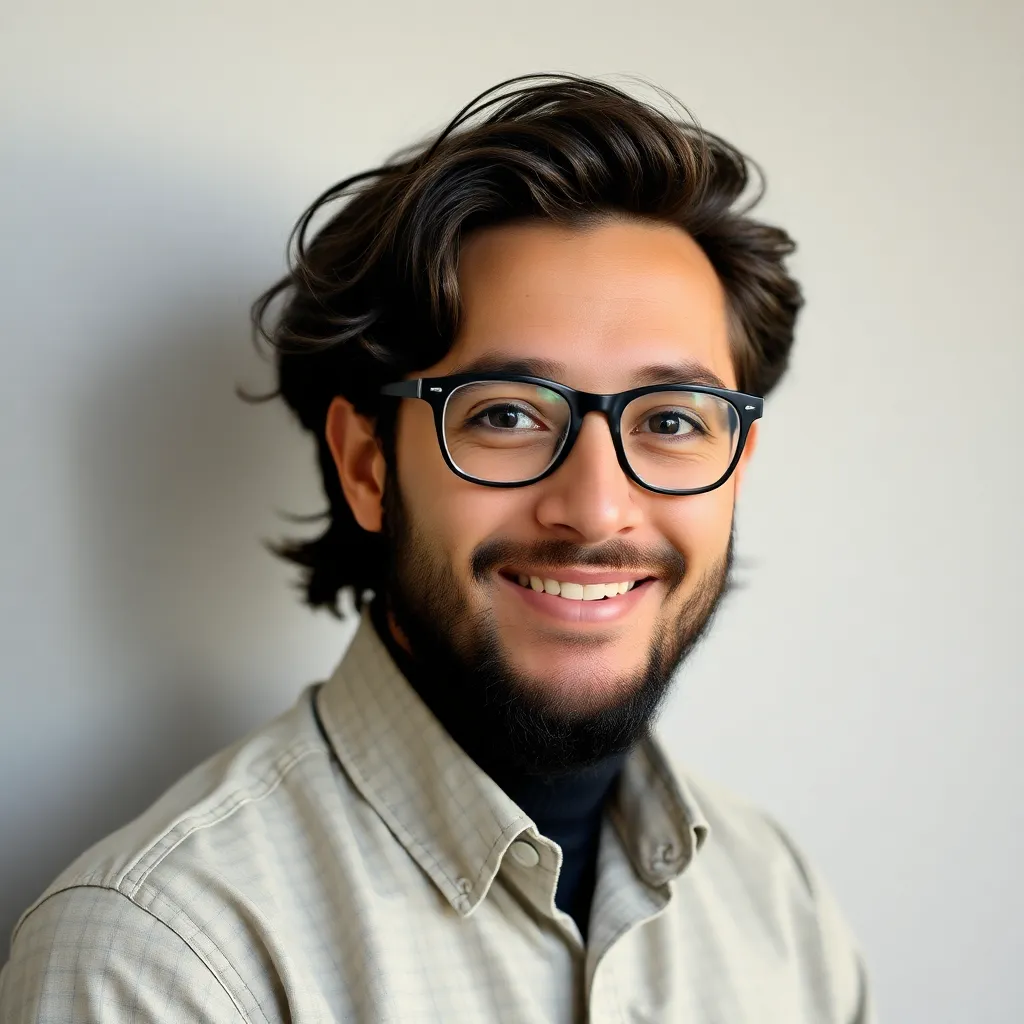
Kalali
Apr 19, 2025 · 8 min read
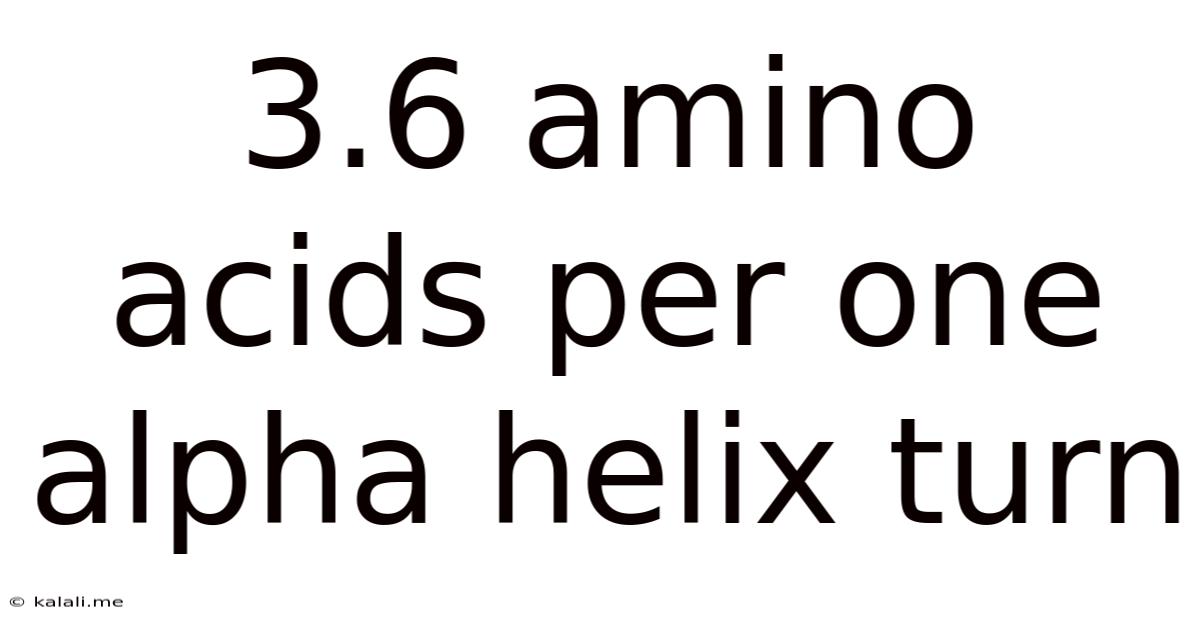
Table of Contents
3.6 Amino Acids Per Alpha Helix Turn: A Deep Dive into Protein Structure and Function
Meta Description: This comprehensive article explores the fundamental concept of 3.6 amino acids per turn in alpha helices, delving into the structural basis, its impact on protein function, and the implications of deviations from this ideal number. We'll examine the underlying physics, relevant calculations, and the role of amino acid side chains in helix stability.
Proteins, the workhorses of life, perform a vast array of functions, from catalyzing biochemical reactions to providing structural support. Their ability to carry out these diverse roles hinges critically on their three-dimensional structures, which are intricately determined by the sequence of amino acids they contain. One of the most common secondary structural motifs found in proteins is the alpha helix, a right-handed coiled structure stabilized by hydrogen bonds. A key characteristic of this helix is its 3.6 amino acid residues per turn. This seemingly simple number holds profound implications for protein structure, stability, and ultimately, function. This article delves deep into this fundamental aspect of protein biochemistry, exploring its significance and the nuances that surround it.
The Structural Basis of the 3.6 Amino Acid Per Turn Rule
The alpha helix is a remarkably stable structure. Its stability arises from a specific pattern of hydrogen bonding between the carbonyl oxygen of one amino acid and the amide hydrogen of the amino acid four residues down the chain. This creates a regular hydrogen-bonded backbone, forming the characteristic helical structure. The distance along the helix axis between successive amino acid residues is approximately 1.5 Å. The rise per residue (the vertical distance moved along the helix axis per residue) is approximately 1.5 Å, while the pitch (the distance traveled along the helix axis per complete turn) is approximately 5.4 Å (1.5 Å/residue * 3.6 residues/turn).
This results in approximately 3.6 amino acid residues per complete turn. This number is not arbitrary; it’s a consequence of the optimal geometry for hydrogen bonding and the van der Waals interactions between adjacent amino acid side chains. Any deviation from this ideal number can significantly affect the stability and function of the helix.
Factors Influencing Helix Stability and the 3.6 Residue Rule:
Several factors contribute to the stability of the alpha helix and influence the precise number of residues per turn:
-
Hydrogen Bonding: The backbone hydrogen bonds are the primary stabilizing force. The geometry of these bonds is optimized for a 3.6 residue/turn structure. Distortions that disrupt these hydrogen bonds destabilize the helix.
-
Van der Waals Interactions: Interactions between side chains contribute to helix stability. Optimal packing of side chains further supports the 3.6 residue/turn configuration. Steric clashes between bulky side chains can disrupt the helix.
-
Amino Acid Sequence: The identity and sequence of amino acids strongly influence helix formation and stability. Certain amino acids (e.g., alanine, leucine) are helix-formers, while others (e.g., proline, glycine) are helix-breakers. Proline, due to its rigid cyclic structure, disrupts the regular hydrogen bonding pattern. Glycine, being highly flexible, can adopt conformations that destabilize the helix.
-
Electrostatic Interactions: Interactions between charged amino acid side chains can influence helix stability. Repulsive interactions between similarly charged residues can destabilize the helix, while attractive interactions between oppositely charged residues can stabilize it.
Deviations from the 3.6 Residue Per Turn Ideal:
While 3.6 amino acids per turn is a commonly observed and idealized value, it's important to understand that this is an average. The actual number of residues per turn can vary slightly depending on the amino acid sequence, surrounding environment, and interactions with other parts of the protein. Factors such as:
-
Helix Dipole: The alpha helix possesses a significant dipole moment, with the amino-terminus being partially positive and the carboxyl-terminus being partially negative. This dipole can influence the interactions of charged residues with the helix, thereby affecting the number of residues per turn.
-
Solvent Effects: The surrounding solvent environment can also affect helix stability and the number of residues per turn. Hydrophobic interactions between side chains and the solvent can stabilize the helix, while interactions between polar side chains and the solvent can influence helix geometry.
-
Protein-Protein Interactions: Interactions with other proteins or molecules can induce conformational changes in the helix, leading to variations in the number of residues per turn.
These deviations, however subtle, can have significant functional consequences. For instance, a slight alteration in the helix pitch can affect the binding affinity of a protein for its ligand or its interaction with another protein.
Calculating Turns and Residues:
Understanding the relationship between helix length and number of turns requires straightforward calculation. If we know the number of residues (n) in an alpha helix, we can approximate the number of turns (T) using the formula:
T ≈ n / 3.6
Conversely, if we know the number of turns, we can estimate the number of residues:
n ≈ T * 3.6
It's crucial to remember that these are approximations. The actual number of turns can vary slightly due to the factors discussed above.
The Role of Amino Acid Side Chains in Helix Stability:
The nature of the amino acid side chains plays a crucial role in determining helix stability. Certain amino acids are strong helix formers, exhibiting a high propensity to adopt helical conformations. These typically include:
-
Alanine (Ala): Its small, nonpolar side chain allows for optimal packing within the helix.
-
Leucine (Leu): Its hydrophobic side chain contributes to helix stability through hydrophobic interactions.
-
Glutamic acid (Glu) and Aspartic acid (Asp): While charged, these amino acids can contribute to helix stability, especially if their charges are appropriately positioned to interact favorably with other residues in the helix or in the surrounding environment.
In contrast, some amino acids are helix breakers, disrupting the regular hydrogen bonding pattern and destabilizing the helix. These include:
-
Proline (Pro): Its rigid cyclic structure prevents the formation of the typical hydrogen bond in the alpha helix backbone.
-
Glycine (Gly): Its high flexibility allows for a range of conformations that can destabilize the helix.
The distribution and arrangement of these helix-forming and helix-breaking residues in the amino acid sequence greatly influence the stability and overall structure of the alpha helix. The interplay of these effects governs the subtle variations observed from the ideal 3.6 amino acids per turn.
Implications for Protein Function:
The precise number of amino acids per turn in an alpha helix is not merely a structural curiosity; it has profound implications for protein function. Any deviation, however small, can significantly alter the protein's overall structure and consequently its ability to interact with other molecules or perform its biological role.
For instance:
-
Enzyme Active Sites: Alpha helices often form part of enzyme active sites. The precise geometry of the helix, determined by the number of residues per turn, is crucial for the correct positioning of catalytic residues and substrate binding. Even small deviations can drastically affect enzymatic activity.
-
Protein-Protein Interactions: Many proteins interact with each other through specific interfaces involving alpha helices. The geometry of these helices is crucial for the specificity and affinity of these interactions. Changes in the number of residues per turn can disrupt these interactions, potentially leading to impaired protein function.
-
Membrane Proteins: Many membrane proteins contain transmembrane alpha helices. The number of residues per turn affects how these helices span the membrane bilayer. Deviations from the ideal can alter the protein's orientation and function within the membrane.
-
Signal Transduction: Many signal transduction proteins rely on conformational changes involving alpha helices to transmit signals. The precise geometry of the helices is crucial for these conformational changes to occur correctly.
Advanced Techniques for Studying Alpha Helices:
Various advanced techniques are used to study alpha helices and their structural features:
-
X-ray Crystallography: Provides high-resolution structural information, allowing precise measurement of the number of residues per turn in an alpha helix.
-
Nuclear Magnetic Resonance (NMR) Spectroscopy: A powerful technique for studying protein structure in solution, providing information about the dynamics and flexibility of alpha helices.
-
Circular Dichroism (CD) Spectroscopy: A sensitive technique for detecting secondary structure elements, including alpha helices. CD can be used to quantitatively assess the alpha-helical content of a protein and to monitor changes in helical structure.
-
Molecular Dynamics (MD) Simulations: Computational methods that simulate the behavior of proteins over time, providing insights into the factors that influence alpha-helix stability and dynamics.
Conclusion:
The 3.6 amino acids per turn rule for alpha helices is a cornerstone of protein structure and function. While an idealization, it represents a crucial structural parameter influencing numerous aspects of protein behavior. Understanding the underlying principles, the factors influencing deviations from this ideal, and the functional implications of such variations is critical for comprehending the complexities of protein biology. Further research into the subtle nuances of alpha-helix structure promises to unveil deeper insights into the intricacies of protein folding, stability, and their remarkable roles in all living systems. The ongoing development and refinement of experimental and computational techniques will continue to provide a more detailed understanding of these crucial aspects of protein biology.
Latest Posts
Latest Posts
-
Least Common Multiple 4 And 8
Apr 22, 2025
-
How Many Hours Is 205 Minutes
Apr 22, 2025
-
What Is 85 Degrees In Celsius
Apr 22, 2025
-
What Percentage Is 7 Out Of 10
Apr 22, 2025
-
Convert 59 Degrees Fahrenheit To Celsius
Apr 22, 2025
Related Post
Thank you for visiting our website which covers about 3.6 Amino Acids Per One Alpha Helix Turn . We hope the information provided has been useful to you. Feel free to contact us if you have any questions or need further assistance. See you next time and don't miss to bookmark.