Electron Transport Chain Inputs And Outputs
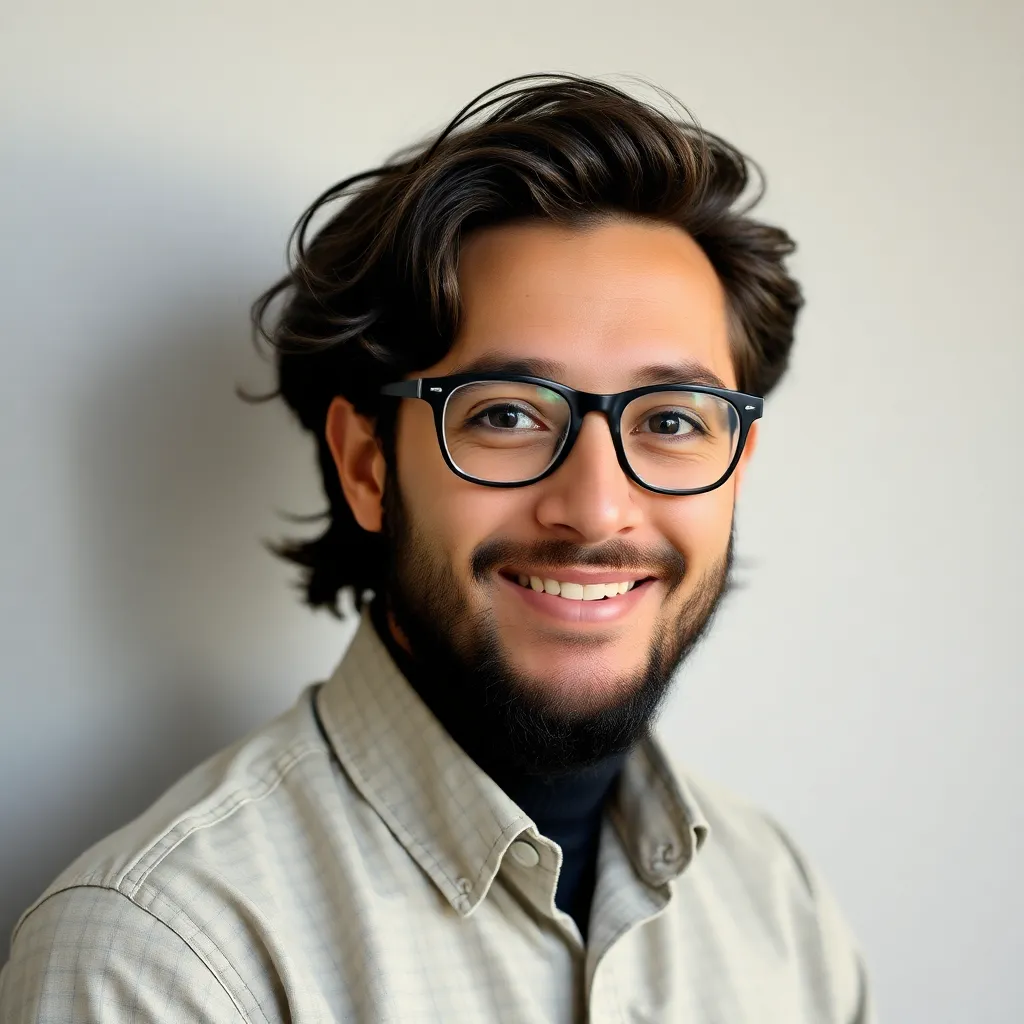
Kalali
Apr 13, 2025 · 7 min read
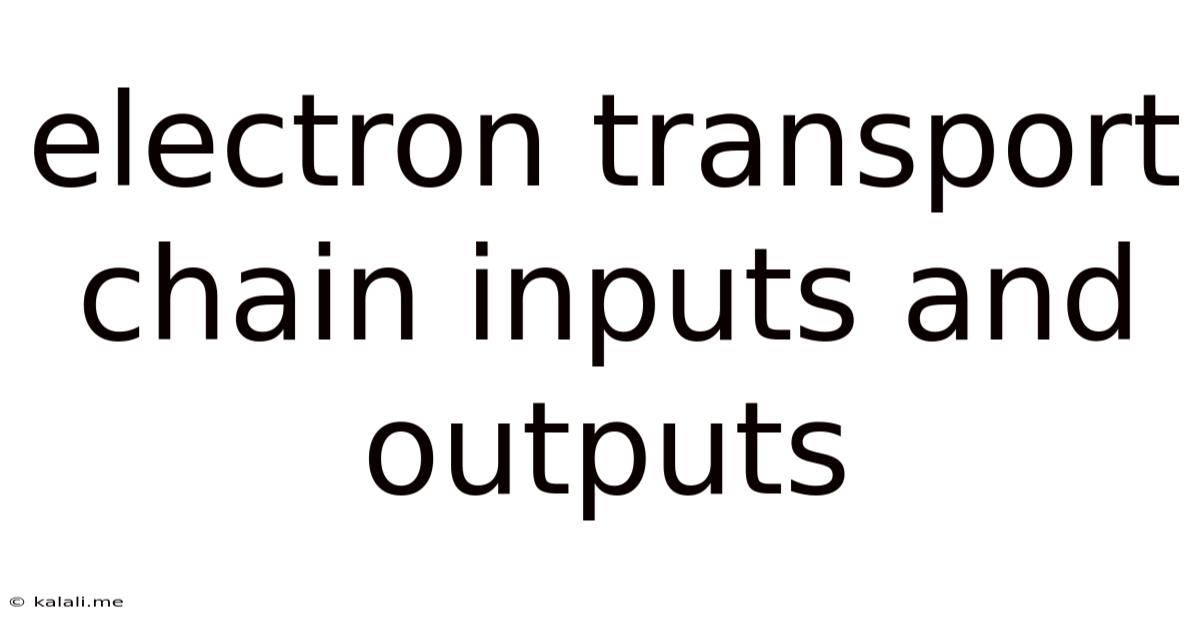
Table of Contents
Electron Transport Chain Inputs and Outputs: A Comprehensive Guide
The electron transport chain (ETC), also known as the respiratory chain, is a series of protein complexes embedded in the inner mitochondrial membrane of eukaryotic cells and the plasma membrane of prokaryotes. This crucial process plays a central role in cellular respiration, converting the chemical energy stored in reduced electron carriers (like NADH and FADH2) into a usable form of energy – ATP (adenosine triphosphate). Understanding the inputs and outputs of the ETC is fundamental to grasping its function and significance in cellular metabolism. This article provides a comprehensive overview of the ETC, detailing its inputs, outputs, and the intricate processes involved.
Meta Description: This in-depth guide explores the electron transport chain (ETC), detailing its crucial inputs (NADH, FADH2, oxygen) and outputs (ATP, water, heat), explaining the process of oxidative phosphorylation and its importance in cellular energy production.
Inputs to the Electron Transport Chain: Fueling the Energy Production
The ETC doesn't work in isolation; it requires specific "fuel" molecules to drive the process of oxidative phosphorylation. These inputs are primarily reduced electron carriers, which deliver high-energy electrons to the chain. Let's examine the key inputs:
1. NADH (Nicotinamide Adenine Dinucleotide): NADH is a major electron carrier generated during glycolysis and the citric acid cycle (Krebs cycle). It carries two high-energy electrons and a proton (H+), representing a significant energy source for the ETC. Each NADH molecule donates its electrons to Complex I of the ETC, initiating the electron transport process. The high energy electrons from NADH have a greater potential energy than those from FADH2, allowing for the production of more ATP molecules per NADH molecule.
2. FADH2 (Flavin Adenine Dinucleotide): FADH2, another crucial electron carrier, is produced during the citric acid cycle. Similar to NADH, it carries two high-energy electrons and two protons. However, FADH2 enters the ETC at Complex II, bypassing Complex I. This bypass results in a slightly lower ATP yield per FADH2 molecule compared to NADH because fewer protons are pumped across the inner mitochondrial membrane. The difference in entry point highlights the nuanced regulation of energy transfer within the cell.
3. Oxygen (O2): Oxygen serves as the final electron acceptor in the ETC. This crucial role explains why aerobic respiration (requiring oxygen) is so much more efficient than anaerobic respiration. Oxygen's high electronegativity allows it to readily accept the electrons passed down the chain, forming water (H2O). Without oxygen, the ETC would become blocked, halting ATP production and leading to cellular dysfunction. The role of oxygen in the ETC is often underestimated, but it's the critical molecule that allows the entire system to function effectively. The lack of a suitable terminal electron acceptor is the reason anaerobic respiration produces far less ATP.
Outputs of the Electron Transport Chain: The Products of Oxidative Phosphorylation
The ETC's primary function is to generate ATP through a process called oxidative phosphorylation. This involves the harnessing of the energy released during electron transport to pump protons (H+) across the inner mitochondrial membrane, creating a proton gradient. This gradient drives ATP synthesis via ATP synthase. The outputs of the ETC include:
1. ATP (Adenosine Triphosphate): The primary output, ATP, is the cell's primary energy currency. The proton gradient generated by the ETC drives ATP synthase, an enzyme that phosphorylates ADP (adenosine diphosphate) to ATP. This process is called chemiosmosis, coupling the electron transport process to ATP synthesis. The exact number of ATP molecules produced per NADH and FADH2 molecule is still debated, with estimates ranging from 2.5 ATP per NADH and 1.5 ATP per FADH2 to slightly lower values due to proton leakages and variations in the efficiency of the process. However, it's clear that a substantial amount of ATP is generated via this process. The yield of ATP directly impacts cellular function, particularly during periods of high energy demand.
2. Water (H2O): As mentioned earlier, oxygen acts as the final electron acceptor in the ETC. When oxygen accepts the electrons, it combines with protons (H+) to form water. This is a crucial byproduct of the process, highlighting the essential role of oxygen in cellular respiration. The formation of water is a crucial step in completing the electron transport chain.
3. Heat: The ETC is not perfectly efficient. Some of the energy released during electron transport is lost as heat. This heat production contributes to the body's overall temperature regulation, particularly in endothermic (warm-blooded) organisms. While heat production is often seen as a byproduct, its contribution to maintaining body temperature shouldn't be ignored, especially in mammals and birds.
The Components of the Electron Transport Chain: A Closer Look
The ETC consists of four major protein complexes (I-IV) embedded in the inner mitochondrial membrane, along with two mobile electron carriers: ubiquinone (CoQ) and cytochrome c. Each complex plays a specific role in the electron transport process:
-
Complex I (NADH dehydrogenase): Receives electrons from NADH and transfers them to ubiquinone. This transfer is coupled with the pumping of protons across the inner mitochondrial membrane.
-
Complex II (succinate dehydrogenase): Receives electrons from FADH2 and transfers them to ubiquinone. Unlike Complex I, it doesn't pump protons.
-
Ubiquinone (CoQ): A lipid-soluble electron carrier that shuttles electrons from Complexes I and II to Complex III.
-
Complex III (cytochrome bc1 complex): Receives electrons from ubiquinone and transfers them to cytochrome c. This process also involves proton pumping.
-
Cytochrome c: A water-soluble electron carrier that shuttles electrons from Complex III to Complex IV.
-
Complex IV (cytochrome c oxidase): Receives electrons from cytochrome c and transfers them to oxygen, the final electron acceptor, forming water. This complex also pumps protons.
The sequential electron transfer through these complexes creates the proton gradient crucial for ATP synthesis via chemiosmosis. Any disruption to this carefully orchestrated process can have serious consequences for cellular energy production.
Regulation of the Electron Transport Chain: Maintaining Cellular Homeostasis
The ETC's activity is tightly regulated to meet the cell's energy demands and maintain cellular homeostasis. Several factors influence its activity:
-
Substrate availability: The availability of NADH and FADH2 directly affects the rate of electron transport. Higher levels of these electron carriers will stimulate the ETC.
-
Oxygen availability: Oxygen is the final electron acceptor, and its availability is crucial for ETC function. Low oxygen levels will inhibit the ETC, leading to a reduction in ATP production.
-
Inhibitors and uncouplers: Various molecules can inhibit or uncouple the ETC. Inhibitors block electron transport at specific points in the chain, while uncouplers disrupt the proton gradient, reducing ATP synthesis but increasing heat production.
Understanding these regulatory mechanisms is crucial for comprehending how cells adapt to changing metabolic conditions and maintain energy balance. The intricate regulation of the ETC ensures that energy production is finely tuned to the cell's needs.
Clinical Significance and Diseases Related to ETC Dysfunction
Disruptions in the ETC's function can have severe consequences, leading to various diseases. Mutations in genes encoding ETC components can result in mitochondrial disorders, characterized by a wide range of symptoms depending on the affected tissues and the severity of the dysfunction. These disorders often affect energy-demanding tissues like the brain, heart, and muscles. Some examples include:
-
Mitochondrial encephalomyopathy, lactic acidosis, and stroke-like episodes (MELAS): A mitochondrial disease associated with mutations in mitochondrial DNA.
-
Myoclonic epilepsy with ragged-red fibers (MERRF): Another mitochondrial disease characterized by myoclonic seizures and ragged-red fibers in muscle biopsies.
-
Leigh syndrome: A neurological disorder affecting the central nervous system.
Furthermore, ETC dysfunction is implicated in various other conditions, including aging, cancer, and neurodegenerative diseases. Research continues to explore the complex relationship between ETC malfunction and these conditions. The ETC is not just a simple energy production system but a vital component of cellular health and function.
Conclusion: The Electron Transport Chain - A Vital Cellular Process
The electron transport chain is a remarkably intricate and efficient system responsible for the majority of ATP production in aerobic organisms. Its inputs – NADH, FADH2, and oxygen – provide the fuel for oxidative phosphorylation, generating ATP, water, and heat as outputs. Understanding the inputs and outputs of the ETC, along with its components, regulation, and clinical significance, is essential for appreciating the complexities of cellular metabolism and its role in maintaining life. Continued research into this critical process will undoubtedly lead to a deeper understanding of cellular function and the development of new therapeutic strategies for mitochondrial diseases.
Latest Posts
Latest Posts
-
How Many Ounces Are In 2 3
Apr 13, 2025
-
What Is The General Form Of A Synthesis Reaction
Apr 13, 2025
-
Least Common Multiple Of 7 And 2
Apr 13, 2025
-
How Many Feet In 1000 Meters
Apr 13, 2025
-
What Is 3 16 As A Decimal
Apr 13, 2025
Related Post
Thank you for visiting our website which covers about Electron Transport Chain Inputs And Outputs . We hope the information provided has been useful to you. Feel free to contact us if you have any questions or need further assistance. See you next time and don't miss to bookmark.