How Do Longitudinal Wave Particles Move
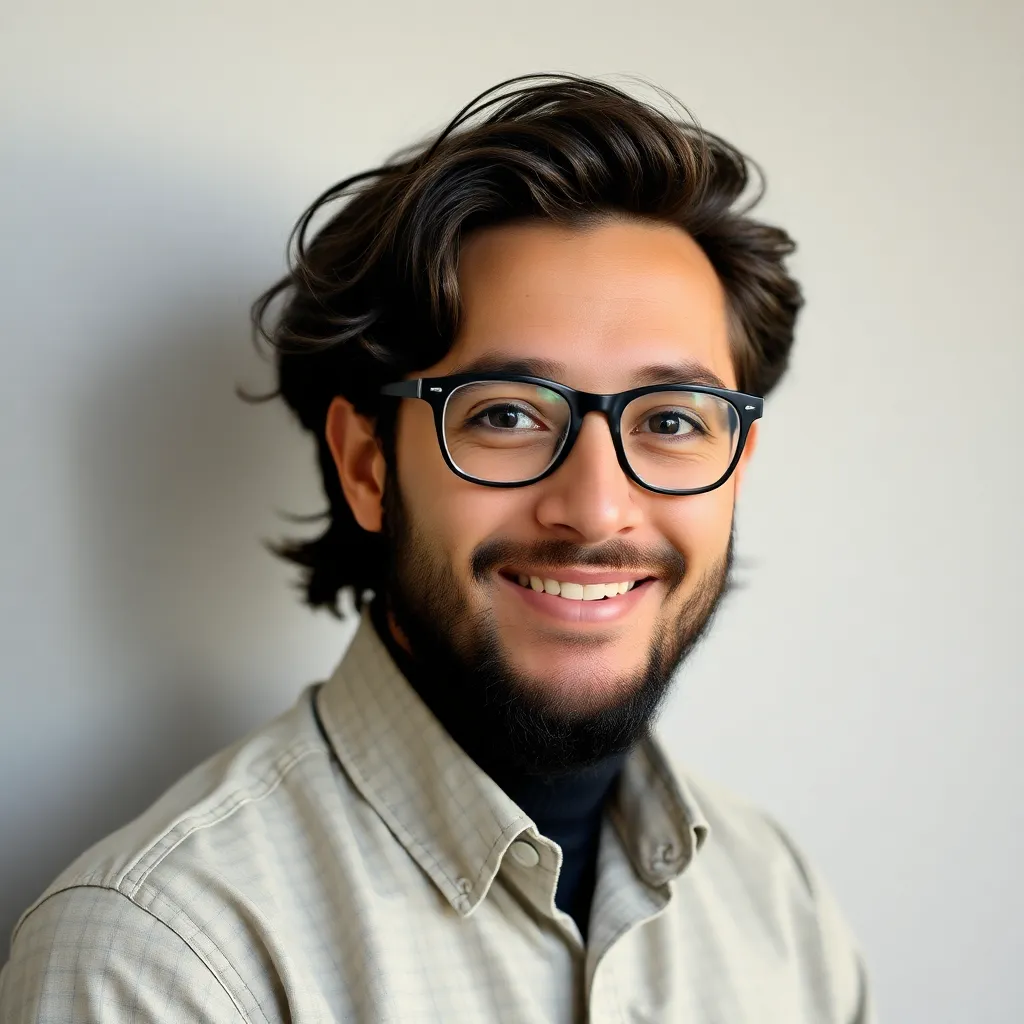
Kalali
Apr 01, 2025 · 6 min read
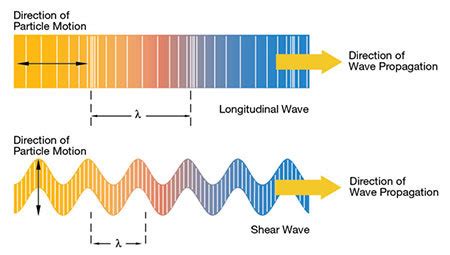
Table of Contents
How Do Longitudinal Wave Particles Move? A Deep Dive into Compression and Rarefaction
Understanding how particles move in a longitudinal wave is crucial to grasping the fundamental principles of wave physics. Unlike transverse waves, where particle oscillation is perpendicular to the wave's direction of travel, longitudinal waves exhibit particle motion parallel to the wave's propagation. This seemingly simple difference has profound implications across various scientific disciplines, from sound propagation to seismic activity. This comprehensive article will delve into the mechanics of longitudinal wave particle movement, exploring the concepts of compression and rarefaction, providing illustrative examples, and addressing common misconceptions.
Understanding the Basics: Longitudinal vs. Transverse Waves
Before diving into the specifics of particle motion, let's establish a clear distinction between longitudinal and transverse waves. Both are types of mechanical waves, requiring a medium (like air, water, or a solid) for propagation.
-
Transverse Waves: In transverse waves, the particles of the medium oscillate perpendicular to the direction of energy transfer. Think of a wave on a string; the string particles move up and down, while the wave travels horizontally. Examples include light waves (electromagnetic, but conceptually similar) and waves on the surface of water.
-
Longitudinal Waves: In longitudinal waves, the particles of the medium oscillate parallel to the direction of energy transfer. This oscillation involves a rhythmic compression and rarefaction of the medium. Imagine pushing and pulling a spring; the coils move back and forth along the spring's length, representing the particle motion, while the compression and rarefaction wave travels along the spring. Sound waves are a prime example of longitudinal waves.
The Dance of Compression and Rarefaction: The Heart of Longitudinal Wave Motion
The characteristic movement of particles in a longitudinal wave is best described by the concepts of compression and rarefaction.
Compression: The Squeeze
When a longitudinal wave propagates through a medium, it creates regions of increased density known as compressions. In these regions, the particles are pushed closer together than their equilibrium positions. Imagine squeezing a spring; the coils become densely packed in the compressed region. This compression represents a high-pressure zone in the medium. The energy of the wave is transferred through this increased density.
Rarefaction: The Stretch
Following a compression, the wave creates a region of decreased density called a rarefaction. Here, the particles are pulled farther apart than their equilibrium positions, resulting in a lower pressure zone. Returning to our spring analogy, after the compression, the coils are stretched further apart in the rarefaction region. This expansion follows the compression, creating the oscillating pattern of the longitudinal wave.
Visualizing Particle Motion: A Step-by-Step Guide
Let's visualize this particle movement in a simplified model. Imagine a line of equally spaced particles representing a medium at rest:
o o o o o o o o o o
Now, let's introduce a longitudinal wave propagating from left to right. The wave will cause the particles to move back and forth along the same line:
-
Compression: The first particle moves to the right, compressing against its neighbor. The compression continues down the line.
o o o o o o o o o
➡️o o o o o o o o o
➡️o o o o o o o o
-
Rarefaction: As the wave progresses, the particles begin to move back to their equilibrium positions, creating a region of rarefaction behind the compression.
o o o o o o o o o o
➡️o o o o o o o o o
➡️o o o o o o o o o
This cycle of compression and rarefaction repeats continuously as the wave propagates through the medium. The particles themselves do not travel with the wave; instead, they oscillate around their equilibrium positions. It's the pattern of compression and rarefaction that moves, transferring energy through the medium.
Examples of Longitudinal Waves in Action
Longitudinal waves are ubiquitous in our world. Here are some key examples:
-
Sound Waves: Sound is arguably the most familiar example of a longitudinal wave. When an object vibrates (like a vocal cord or a musical instrument), it creates compressions and rarefactions in the surrounding air. These compressions and rarefactions travel outwards as sound waves, eventually reaching our ears, where they are interpreted as sound. The frequency of these compressions and rarefactions determines the pitch of the sound, while the amplitude (the size of the compressions and rarefactions) determines the loudness.
-
Seismic P-waves: During an earthquake, two main types of seismic waves are generated: P-waves (primary waves) and S-waves (secondary waves). P-waves are longitudinal waves that travel through the Earth's interior, causing the ground to alternately compress and rarefy. These waves are faster than S-waves and are the first to reach seismograph stations after an earthquake.
-
Ultrasound: Medical ultrasound uses high-frequency sound waves to create images of internal organs. The reflection of these longitudinal waves from different tissues provides information about the tissues' density and structure.
Factors Affecting Longitudinal Wave Propagation
Several factors influence the speed and characteristics of longitudinal wave propagation:
-
Medium Density: The speed of a longitudinal wave is generally slower in denser media. This is because particles in a denser medium are more tightly packed, making it more difficult for the wave to propagate.
-
Medium Elasticity: The elasticity of the medium affects the speed of the wave. A more elastic medium (one that readily returns to its original shape after deformation) allows for faster wave propagation.
-
Temperature: In many cases, the temperature of the medium affects the speed of sound waves. For instance, sound travels faster in warmer air than in colder air because warmer air has higher molecular kinetic energy.
Addressing Common Misconceptions
A common misunderstanding is that particles in a longitudinal wave move along with the wave itself. It's crucial to remember that the particles oscillate around their equilibrium positions, while the wave's energy propagates forward. The particles don't travel from the source to the destination; the energy does.
Another misconception is that only gases can support longitudinal waves. While gases are excellent mediums for longitudinal waves, solids and liquids also transmit longitudinal waves, often with greater speed than in gases.
Conclusion: A Deeper Understanding of Longitudinal Wave Motion
Understanding how particles move in a longitudinal wave – the interplay of compression and rarefaction – is fundamental to comprehending wave phenomena. From the sounds we hear to the seismic waves that shake the Earth, the principles discussed here are essential to various fields of science and engineering. By grasping the intricacies of this particle motion, we gain a more profound appreciation for the unseen forces that shape our world. This detailed exploration provides a solid foundation for further study in acoustics, seismology, and other related disciplines. The ability to visualize and explain this movement is critical for effective communication and problem-solving in these fields. Further research into specific mediums and wave interactions will deepen this understanding even further.
Latest Posts
Latest Posts
-
42 Feet Is How Many Meters
Apr 02, 2025
-
What Is 20 Out Of 25 As A Percentage
Apr 02, 2025
-
How Many Grams In 1 4 Ounces
Apr 02, 2025
-
How Many Centimeters Are In 20 Meters
Apr 02, 2025
-
5 1 2 Pulgadas A Centimetros
Apr 02, 2025
Related Post
Thank you for visiting our website which covers about How Do Longitudinal Wave Particles Move . We hope the information provided has been useful to you. Feel free to contact us if you have any questions or need further assistance. See you next time and don't miss to bookmark.