How Do The Bases Bond Together
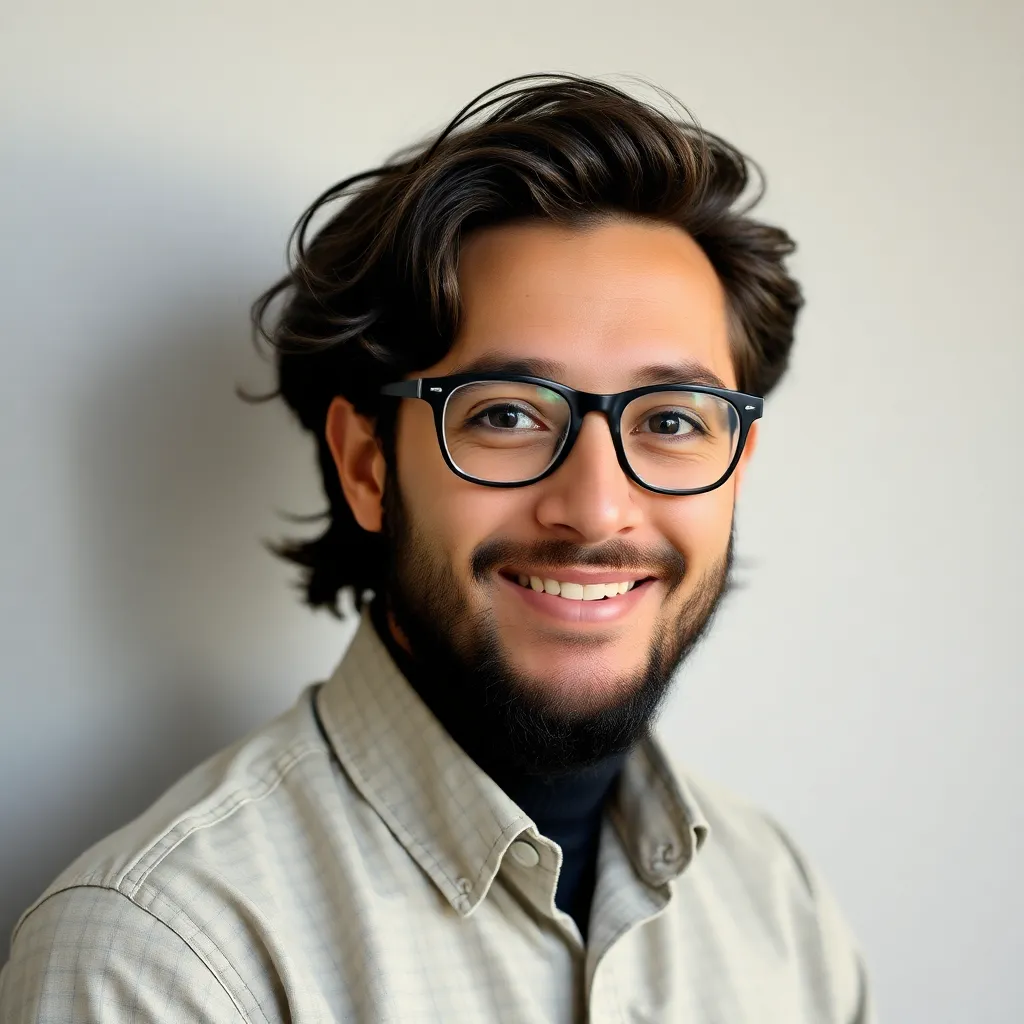
Kalali
Apr 05, 2025 · 6 min read
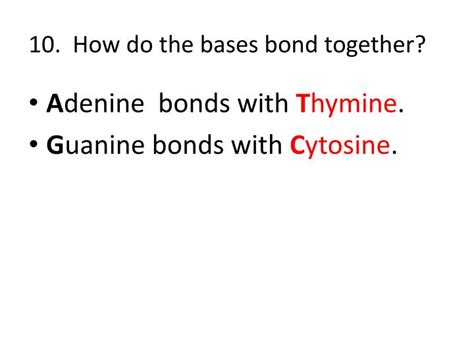
Table of Contents
How Do the Bases Bond Together? Understanding Base Pairing in Nucleic Acids
The intricate dance of life hinges on the precise pairing of nitrogenous bases within the DNA and RNA molecules. This seemingly simple interaction – the bonding between adenine (A), guanine (G), cytosine (C), and thymine (T) – forms the very foundation of heredity, genetic information storage, and protein synthesis. Understanding how these bases bond together is crucial to comprehending the complexities of molecular biology and the mechanisms that drive life itself.
The Players: Purines and Pyrimidines
Before diving into the bonding mechanisms, let's introduce the key players: the nitrogenous bases. These molecules are categorized into two groups based on their chemical structure:
Purines: Adenine (A) and Guanine (G)
Purines are characterized by their double-ring structure, consisting of a six-membered ring fused to a five-membered ring. Both adenine and guanine fall into this category. The specific arrangement of nitrogen and carbon atoms within these rings dictates their bonding capabilities.
Pyrimidines: Cytosine (C), Thymine (T), and Uracil (U)
Pyrimidines, in contrast, possess a single six-membered ring structure. Cytosine, thymine, and uracil (found in RNA instead of thymine) are the pyrimidine bases. Their single-ring structure complements the purines in forming the stable base pairs crucial for nucleic acid structure.
The Bonds: Hydrogen Bonds and Base Pairing
The interaction between purines and pyrimidines isn't a random affair. The bases bond together through a specific type of weak chemical bond called a hydrogen bond. This bond forms between a hydrogen atom covalently bonded to an electronegative atom (like oxygen or nitrogen) and another electronegative atom. While individually weak, the cumulative effect of multiple hydrogen bonds provides the stability required for the double helix structure of DNA and the various conformations of RNA.
Chargaff's Rules and Complementary Base Pairing
The specific pairing of bases is governed by Chargaff's rules, which state that in any DNA molecule, the amount of adenine (A) is equal to the amount of thymine (T), and the amount of guanine (G) is equal to the amount of cytosine (C). This equality stems from the specific hydrogen bonding patterns between the bases.
-
Adenine (A) and Thymine (T) Pairing: Adenine and thymine form two hydrogen bonds. A hydrogen atom from the amino group of adenine forms a hydrogen bond with an oxygen atom in thymine. Another hydrogen bond forms between a nitrogen atom in adenine and another nitrogen atom in thymine. This double hydrogen bond is relatively strong, contributing to the stability of the DNA double helix.
-
Guanine (G) and Cytosine (C) Pairing: Guanine and cytosine form three hydrogen bonds. A hydrogen bond forms between a nitrogen atom in guanine and an oxygen atom in cytosine. Two additional hydrogen bonds occur between a nitrogen atom in guanine and two nitrogen atoms in cytosine. The triple hydrogen bond between G and C makes this base pair stronger than the A-T pair. This difference in bonding strength contributes to the variations in DNA stability and melting temperature.
The Importance of Base Pairing Geometry
The hydrogen bonding between bases isn't just about the number of bonds; it's also about the geometry. The specific arrangement of atoms in the purines and pyrimidines allows for the formation of hydrogen bonds only between specific base pairs (A-T and G-C). The shape and size of the bases are crucial in fitting together accurately within the double helix. An A-C or a G-T pairing would disrupt the overall structure of the DNA double helix, leading to instability and potential errors in DNA replication and transcription.
Base Pairing in DNA: The Double Helix
The base pairing rules are the cornerstone of the iconic double helix structure of DNA. The two strands of DNA are antiparallel (running in opposite directions), and the bases are stacked internally, forming the "rungs" of the ladder-like structure. The sugar-phosphate backbone forms the sides of the ladder. The specific A-T and G-C pairings ensure that the two strands fit together snugly, maintaining the consistent diameter of the double helix.
The Stability of the DNA Double Helix
The stability of the DNA double helix is directly related to the strength of the hydrogen bonds between base pairs. The collective strength of these bonds, along with other interactions like van der Waals forces and base stacking interactions, helps maintain the double helix's integrity. This stability is vital for preserving the genetic information encoded within the DNA sequence.
Base Pairing in RNA: Variations and Functions
While the principle of base pairing is similar in RNA, there are some key differences. RNA typically exists as a single-stranded molecule, although it can fold into complex three-dimensional structures through intramolecular base pairing. Furthermore, RNA uses uracil (U) instead of thymine (T).
Uracil (U) in RNA: Pairing with Adenine (A)
Uracil, like thymine, pairs with adenine through two hydrogen bonds. The absence of a methyl group on uracil compared to thymine doesn't significantly alter the bonding properties in this pairing.
RNA Secondary Structures: Stem-loops and Hairpins
The single-stranded nature of RNA allows for the formation of various secondary structures through intramolecular base pairing. This means that different parts of the same RNA molecule can pair with each other, creating loops, hairpins, and other complex folding patterns. These structures are crucial for the functionality of many RNA molecules, including tRNA (transfer RNA) and rRNA (ribosomal RNA).
The Implications of Base Pairing Errors
The accuracy of base pairing is vital for the proper functioning of genetic processes. Errors in base pairing during DNA replication can lead to mutations, potentially causing diseases or genetic disorders. The cellular machinery, including DNA polymerase and various repair mechanisms, has evolved to minimize the occurrence of such errors. However, occasional errors do occur, contributing to the genetic diversity within populations.
Conclusion: A Fundamental Interaction
The way the bases bond together—through specific hydrogen bonding patterns—is a fundamental aspect of molecular biology. This seemingly simple interaction underlies the complex mechanisms of DNA replication, transcription, and translation, which drive the expression of genetic information and the propagation of life. Understanding the intricacies of base pairing is key to comprehending the fundamental processes that shape the living world. The specificity and stability afforded by these interactions highlight the elegance and precision of biological systems. Further research continues to unravel the finer details of base pairing, including the influence of the surrounding environment and the role of various proteins in modulating these interactions. The ongoing exploration of these fundamental principles promises to further our understanding of life itself.
Latest Posts
Latest Posts
-
48 Inches Equals How Many Feet
Apr 06, 2025
-
105 Inches Is How Many Feet
Apr 06, 2025
-
220 Out Of 250 As A Percentage
Apr 06, 2025
-
What Is 2 5 As A Fraction
Apr 06, 2025
-
21 Is 75 Of What Number
Apr 06, 2025
Related Post
Thank you for visiting our website which covers about How Do The Bases Bond Together . We hope the information provided has been useful to you. Feel free to contact us if you have any questions or need further assistance. See you next time and don't miss to bookmark.