How Is Protein Structure Involved In Enzyme Specificity
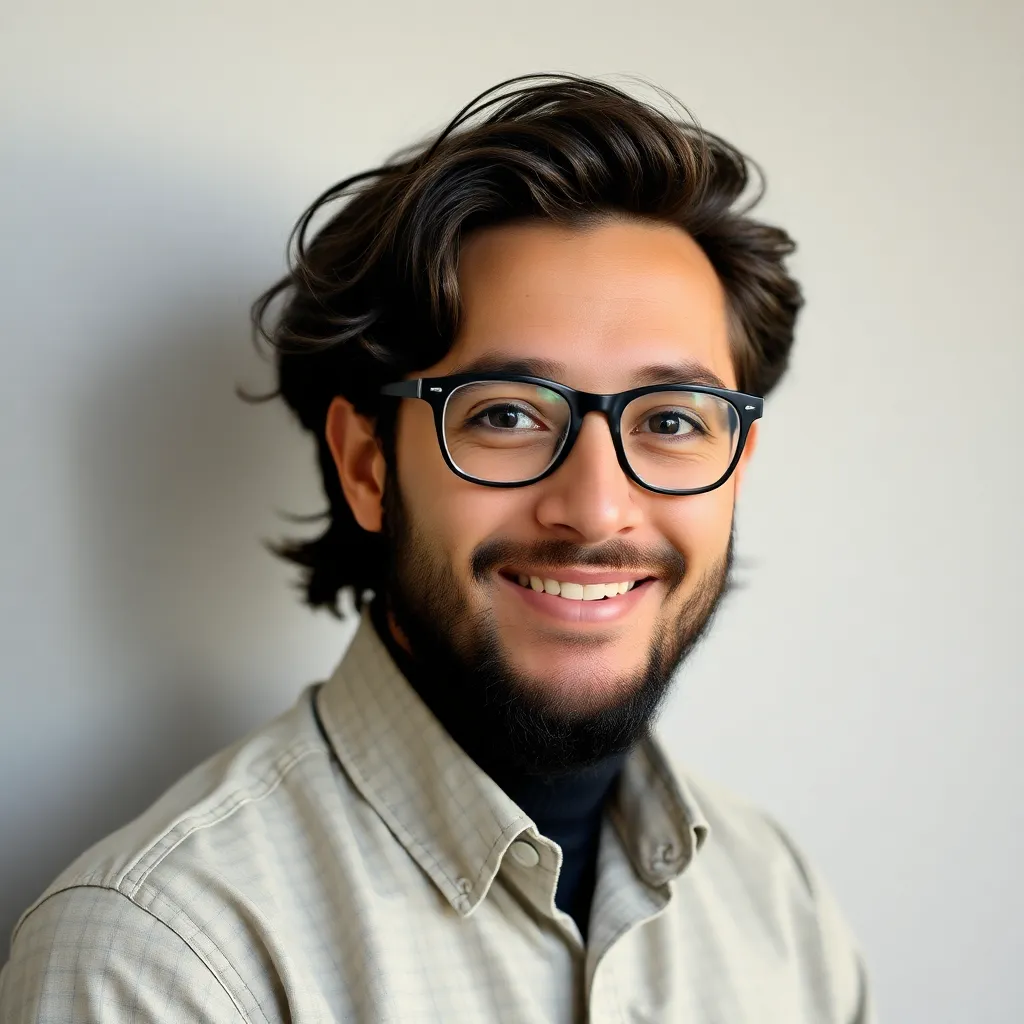
Kalali
Mar 27, 2025 · 6 min read
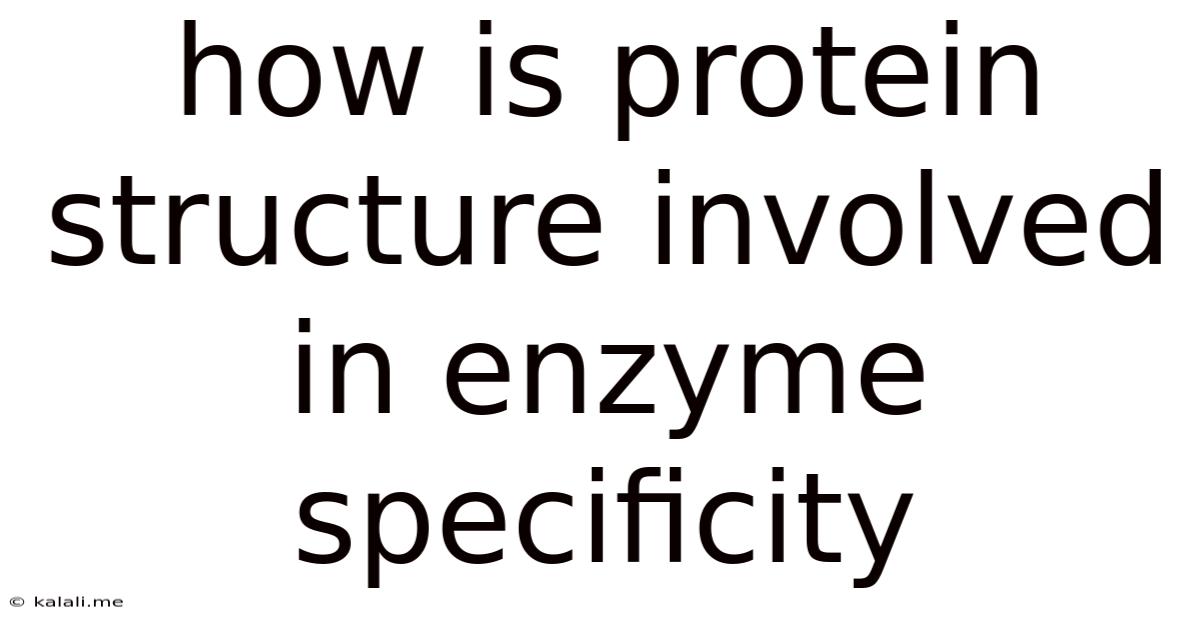
Table of Contents
How is Protein Structure Involved in Enzyme Specificity?
Enzymes are biological catalysts that accelerate the rate of virtually all chemical reactions within cells. Their remarkable efficiency and specificity are intrinsically linked to their intricate three-dimensional structures. Understanding how protein structure dictates enzyme specificity is crucial to comprehending the fundamental mechanisms of life and developing novel therapeutic strategies. This article will delve deep into the relationship between protein structure and enzyme specificity, exploring the various levels of protein organization and their contributions to the precise recognition and binding of substrates.
The Four Levels of Protein Structure: A Foundation for Specificity
Protein structure is hierarchical, encompassing four distinct levels: primary, secondary, tertiary, and quaternary. Each level contributes to the overall three-dimensional shape of the enzyme, influencing its ability to interact with specific substrates.
1. Primary Structure: The Amino Acid Sequence
The primary structure of a protein is simply the linear sequence of amino acids linked together by peptide bonds. This sequence, dictated by the genetic code, is the blueprint for all higher levels of structure. The specific order of amino acids is crucial because each amino acid possesses unique chemical properties – some are hydrophobic, others hydrophilic, some are charged, and others are polar. This diversity in chemical properties directly impacts how the protein folds and ultimately influences its interactions with other molecules, including substrates. Even a single amino acid substitution can drastically alter the protein's function and specificity.
2. Secondary Structure: Local Folding Patterns
The secondary structure refers to local folding patterns within the polypeptide chain stabilized by hydrogen bonds between the backbone atoms. Common secondary structures include α-helices and β-sheets. These structures are not random; they are determined by the interactions between nearby amino acid residues. For example, α-helices are favored by amino acids with small side chains, while β-sheets are often formed by amino acids with alternating hydrophobic and hydrophilic side chains. The arrangement of these secondary structures within a protein significantly contributes to the formation of the active site and the overall shape of the enzyme, hence influencing substrate binding.
3. Tertiary Structure: The 3D Arrangement
The tertiary structure represents the overall three-dimensional arrangement of the polypeptide chain, encompassing all secondary structure elements. This level of structure is primarily determined by interactions between the side chains of amino acids that are distant in the primary sequence. These interactions include:
- Hydrophobic interactions: Nonpolar side chains cluster together in the protein's interior, minimizing their contact with water.
- Hydrogen bonds: Hydrogen bonds form between polar side chains, further stabilizing the protein's structure.
- Ionic interactions (salt bridges): Electrostatic interactions between charged side chains.
- Disulfide bonds: Covalent bonds between cysteine residues, creating strong links within the protein.
The tertiary structure creates a unique three-dimensional shape, including the formation of the active site. The active site is a specific region within the enzyme that binds the substrate and catalyzes the reaction. The precise arrangement of amino acid side chains within the active site is critical for substrate binding and catalysis.
4. Quaternary Structure: Multiple Polypeptide Chains
Some enzymes consist of multiple polypeptide chains, each with its own tertiary structure. The arrangement of these chains forms the quaternary structure. The interactions between subunits are similar to those involved in tertiary structure formation, including hydrophobic interactions, hydrogen bonds, ionic interactions, and disulfide bonds. The quaternary structure can significantly enhance enzyme activity and specificity, allowing for cooperative substrate binding or allosteric regulation.
The Active Site: The Heart of Enzyme Specificity
The active site is a cleft or pocket on the enzyme surface formed by the precise arrangement of amino acid residues from different parts of the polypeptide chain. Its three-dimensional structure is perfectly tailored to accommodate the substrate(s). The specificity of an enzyme is largely determined by the shape and chemical properties of its active site. This principle is often described as the lock-and-key model and the induced-fit model.
Lock-and-Key Model: A Simple Analogy
The lock-and-key model proposes that the active site has a rigid, pre-formed shape that is complementary to the shape of the substrate. The substrate fits perfectly into the active site like a key into a lock, leading to the formation of an enzyme-substrate complex. While a useful simplification, this model doesn't fully explain the flexibility observed in many enzyme-substrate interactions.
Induced-Fit Model: A More Realistic Picture
The induced-fit model provides a more accurate description of enzyme-substrate interactions. This model suggests that the active site is flexible and undergoes a conformational change upon substrate binding. The substrate binding induces a change in the enzyme's shape, creating a more precise fit and optimizing the orientation of catalytic residues for efficient catalysis. This conformational change enhances the enzyme's specificity by ensuring that only the correct substrate can induce the necessary changes for catalysis.
Factors Contributing to Enzyme Specificity
Besides the active site's structure, several other factors contribute to the remarkable specificity of enzymes:
- Shape complementarity: The shape of the active site must be compatible with the shape of the substrate. This includes both the overall shape and the specific orientation of functional groups on the substrate.
- Charge interactions: Electrostatic interactions between charged residues in the active site and charged groups on the substrate contribute to binding specificity.
- Hydrogen bonds: Hydrogen bonds between polar residues in the active site and polar groups on the substrate further enhance binding specificity.
- Hydrophobic interactions: Hydrophobic interactions between nonpolar residues in the active site and nonpolar groups on the substrate can also contribute to binding.
- Transition state stabilization: Enzymes often bind to the transition state of the reaction more tightly than to the substrate or product. This stabilization lowers the activation energy and increases the rate of the reaction. This tight binding is often very specific.
Examples of Enzyme Specificity Linked to Structure
Numerous examples illustrate the critical role of protein structure in enzyme specificity:
- Chymotrypsin: This protease cleaves peptide bonds adjacent to large hydrophobic amino acid residues. Its active site contains a hydrophobic pocket that specifically accommodates these residues.
- Hexokinase: This enzyme phosphorylates hexoses. Its active site undergoes a significant conformational change upon glucose binding, wrapping around the substrate and excluding water molecules.
- Lysozyme: This enzyme hydrolyzes the β-(1,4)-glycosidic bonds in peptidoglycans, a crucial component of bacterial cell walls. The active site of lysozyme is shaped to specifically bind to the hexasaccharide substrate.
Conclusion: A Dynamic Interplay
Enzyme specificity is a remarkable phenomenon driven by the intricate interplay between the enzyme's three-dimensional structure and the substrate's properties. The precise arrangement of amino acids in the active site, governed by the primary, secondary, tertiary, and quaternary structures of the enzyme, dictates its ability to selectively bind and catalyze the transformation of specific molecules. The induced-fit model highlights the dynamic nature of this interaction, where the enzyme actively participates in shaping the substrate for optimal catalysis. A profound understanding of this relationship is crucial for advancements in various fields, including drug design, metabolic engineering, and the development of novel biotechnologies. Future research will undoubtedly continue to unveil the complexities and subtleties of enzyme-substrate interactions, enriching our understanding of biological catalysis and its pivotal role in life's processes.
Latest Posts
Latest Posts
-
What Is 90 Percent Of 36
Apr 28, 2025
-
Rotations Common Core Geometry Homework Answers
Apr 28, 2025
-
What Percent Of 36 Is 45
Apr 28, 2025
-
How Many Centimeters Is 63 Inches
Apr 28, 2025
-
How Many Quarts Are In 3 5 Gallons
Apr 28, 2025
Related Post
Thank you for visiting our website which covers about How Is Protein Structure Involved In Enzyme Specificity . We hope the information provided has been useful to you. Feel free to contact us if you have any questions or need further assistance. See you next time and don't miss to bookmark.