In A Longitudinal Wave The Compressions And Rarefactions Travel In
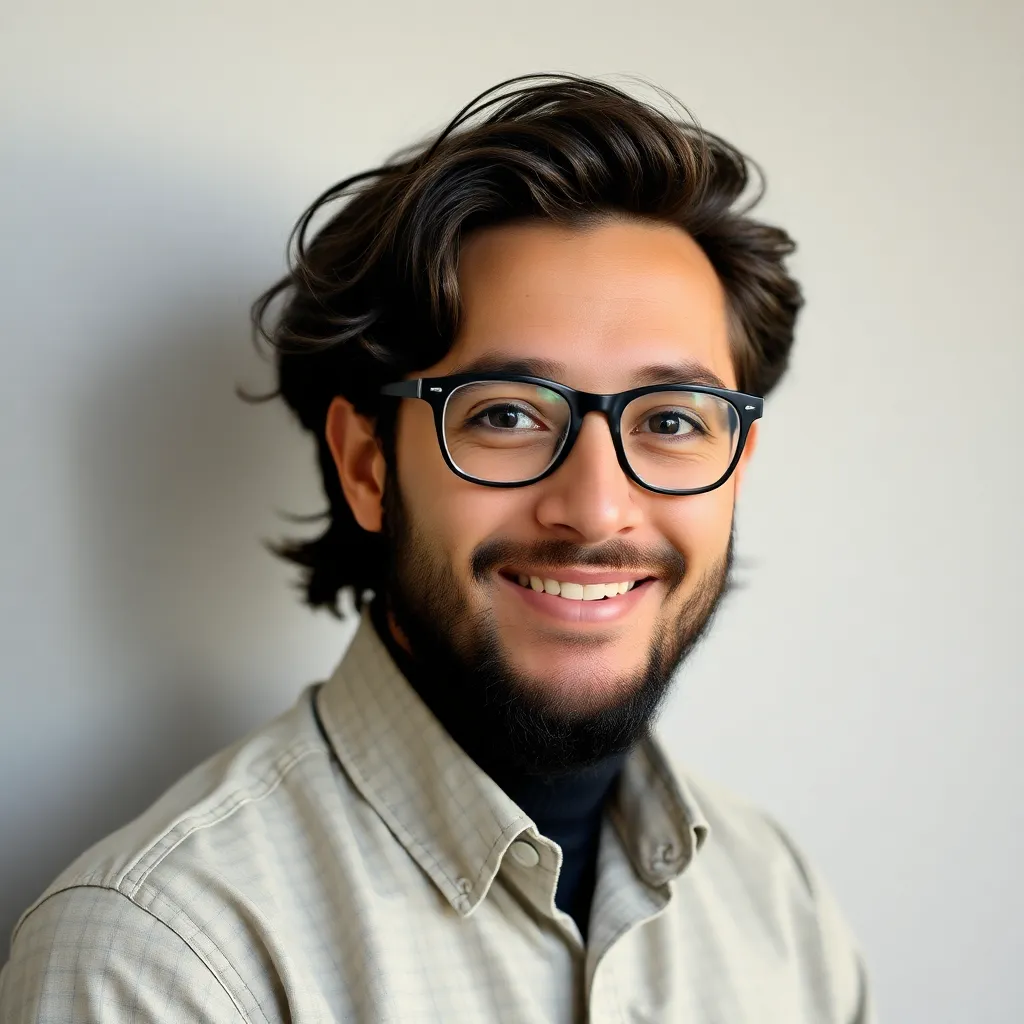
Kalali
Mar 30, 2025 · 5 min read
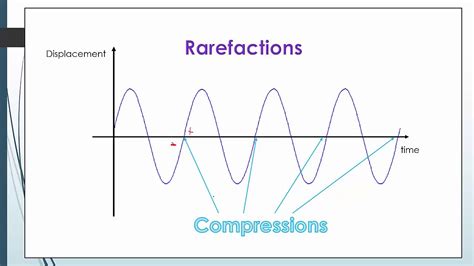
Table of Contents
In a Longitudinal Wave, the Compressions and Rarefactions Travel In… the Same Direction as the Wave!
Understanding wave motion is fundamental to grasping many physical phenomena, from sound to seismic activity. This article delves deep into longitudinal waves, specifically addressing the direction of travel of compressions and rarefactions. We'll explore the concept in detail, providing clear explanations, illustrative examples, and addressing common misconceptions. By the end, you'll have a solid understanding of this crucial aspect of wave physics.
What is a Longitudinal Wave?
Before we dive into the specifics of compression and rarefaction movement, let's define a longitudinal wave. Unlike transverse waves, where the oscillation is perpendicular to the direction of wave propagation (think of a wave on a string), longitudinal waves involve oscillations that occur parallel to the direction of energy transfer. Imagine pushing and pulling a slinky: the coils move back and forth along the same axis as the wave travels.
This parallel oscillation creates distinct regions of:
- Compressions: Areas where the medium (the material the wave travels through) is compressed, resulting in increased density and pressure.
- Rarefactions: Areas where the medium is spread out, resulting in decreased density and pressure.
The Crucial Point: Compressions and Rarefactions Travel in the Same Direction
This is the key concept to grasp: In a longitudinal wave, the compressions and rarefactions travel in the same direction as the wave itself. They are not independent entities moving in different directions; they are integral parts of the wave's propagation. As the wave moves forward, the compressions and rarefactions move with it.
Think of it like a domino effect. When you push the first domino, the disturbance (the wave) travels down the line. Each domino represents a small section of the medium. As the wave passes, each domino moves forward (like the medium in the compression and rarefaction), transferring energy to the next. The disturbance and the movement of the individual dominoes are in the same direction. This analogy perfectly illustrates the movement of compressions and rarefactions in a longitudinal wave.
Visualizing the Movement: Examples and Analogies
Let's solidify this concept with some relatable examples:
-
Sound Waves: Sound travels as a longitudinal wave through air. When you speak, your vocal cords create compressions and rarefactions in the air molecules. These compressions and rarefactions don't travel backward; they travel outward from your mouth, in the same direction as the sound wave. Your ear then detects these variations in pressure, interpreting them as sound.
-
Seismic P-waves: P-waves, or primary waves, are longitudinal seismic waves that travel through the Earth's interior during an earthquake. These waves cause the rocks to compress and expand in the same direction the wave is propagating. This compressional movement allows P-waves to travel faster than other seismic waves.
-
A Spring or Slinky: As mentioned earlier, a spring or slinky is a perfect visual aid. When you push one end of the spring, the compressions (where the coils are close together) and rarefactions (where the coils are spread apart) travel along the spring in the same direction as the initial push.
-
Ultrasound: Medical ultrasound uses high-frequency sound waves (longitudinal waves) to create images of internal organs. The compressions and rarefactions of these sound waves travel through the body, reflecting off tissues to generate the images. Again, the movement of compressions and rarefactions mirrors the direction of the ultrasound wave.
Distinguishing Longitudinal from Transverse Waves
It's essential to distinguish between longitudinal and transverse waves to understand the directionality of compressions and rarefactions. In transverse waves, the oscillation is perpendicular to the direction of energy transfer. Think of a wave on a rope; the rope moves up and down (perpendicular), while the wave travels along the rope. There are no compressions or rarefactions in a transverse wave.
The key difference lies in the direction of particle oscillation relative to the direction of wave propagation. This difference leads to the distinct behavior of compressions and rarefactions in longitudinal waves.
Mathematical Representation of Longitudinal Waves
The behavior of longitudinal waves can be described mathematically using wave equations. The displacement (s) of a particle in the medium at a position (x) and time (t) can be represented by:
s(x,t) = A cos(kx - ωt)
Where:
A
is the amplitude of the wave (maximum displacement)k
is the wave number (2π/λ, where λ is the wavelength)ω
is the angular frequency (2πf, where f is the frequency)
This equation shows how the displacement of particles oscillates along the x-axis (direction of wave propagation). The compressions and rarefactions are represented by regions of positive and negative displacement, respectively, all moving in the positive x-direction.
Applications of Understanding Longitudinal Waves
The understanding of the directional movement of compressions and rarefactions in longitudinal waves is crucial in numerous applications:
-
Acoustics: Designing concert halls, soundproofing materials, and developing audio equipment all rely on a thorough understanding of how sound waves (longitudinal waves) behave.
-
Seismology: Analyzing seismic waves to understand earthquake behavior and predict future events requires knowledge of both P-waves (longitudinal) and S-waves (transverse).
-
Medical Imaging: Ultrasound and other medical imaging techniques utilizing sound waves depend on a precise understanding of how these waves propagate through the body.
-
Materials Science: Studying the behavior of materials under stress and strain often involves analyzing the propagation of longitudinal waves.
Common Misconceptions
It's important to address some common misconceptions surrounding longitudinal waves:
-
Compressions and rarefactions moving independently: As emphasized throughout this article, compressions and rarefactions are not independent entities; they are parts of the same wave, moving together in the same direction.
-
Confusion with transverse waves: The key difference between longitudinal and transverse waves lies in the direction of particle oscillation. Remember, compressions and rarefactions are unique to longitudinal waves.
Conclusion
In a longitudinal wave, the compressions and rarefactions are not independent travelers; they move in the same direction as the wave itself. This fundamental concept is crucial for understanding a wide range of physical phenomena and has significant implications across various scientific and technological fields. By grasping this principle and understanding the differences between longitudinal and transverse waves, you can gain a deeper appreciation of wave motion and its pervasive role in the world around us. This understanding forms the bedrock of numerous advanced concepts in physics and engineering. Continued exploration of wave phenomena will only deepen your understanding of the intricate workings of the universe.
Latest Posts
Latest Posts
-
How Much Is 40 Ml In Cups
Apr 01, 2025
-
5 5 Feet Is How Many Inches
Apr 01, 2025
-
What Is 25 Percent Of 500
Apr 01, 2025
-
How Much Is 60 Oz Water
Apr 01, 2025
-
How Long Is 10km In Miles
Apr 01, 2025
Related Post
Thank you for visiting our website which covers about In A Longitudinal Wave The Compressions And Rarefactions Travel In . We hope the information provided has been useful to you. Feel free to contact us if you have any questions or need further assistance. See you next time and don't miss to bookmark.