In A Longitudinal Wave The Particles Move
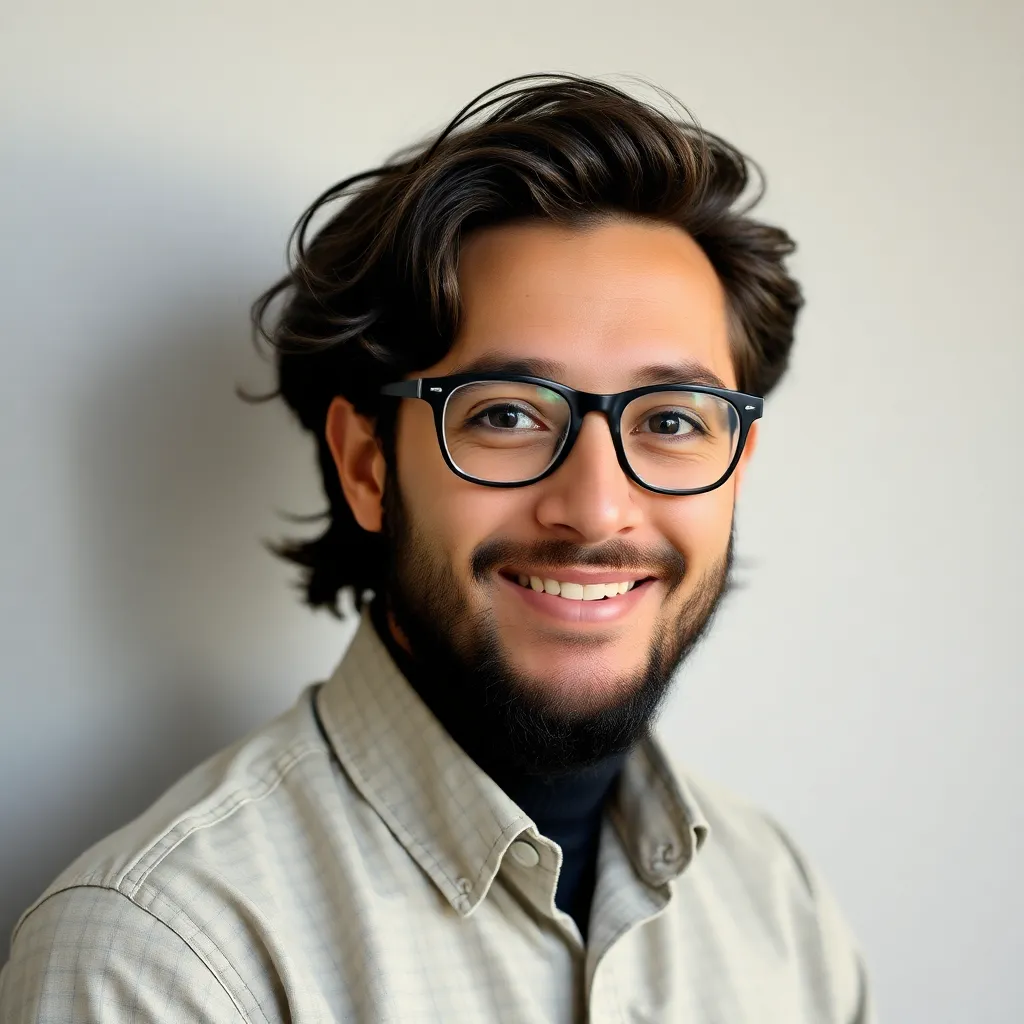
Kalali
Mar 27, 2025 · 6 min read
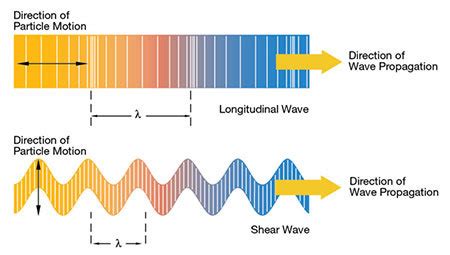
Table of Contents
In a Longitudinal Wave, the Particles Move… Parallel to the Wave's Direction!
Understanding how particles behave in a longitudinal wave is fundamental to grasping wave mechanics. Unlike transverse waves where particle oscillation is perpendicular to the wave's direction, longitudinal waves exhibit a unique particle movement. This article delves deep into this fascinating phenomenon, exploring the mechanics, examples, and applications of longitudinal waves.
Understanding Longitudinal Waves: A Microscopic Perspective
At its core, a longitudinal wave is a type of mechanical wave where the particles of the medium vibrate parallel to the direction of energy transfer. Imagine a slinky: when you push one end, the compression travels down the slinky, not sideways. This compression, and subsequent rarefaction, represents the wave's propagation. The individual coils of the slinky (representing particles in a medium) move back and forth along the slinky's length (the direction of wave propagation). This parallel motion distinguishes longitudinal waves from transverse waves.
The Role of Compression and Rarefaction
The propagation of a longitudinal wave hinges on two key concepts: compression and rarefaction.
-
Compression: This refers to the region in the medium where the particles are crowded together, resulting in a higher density. Think of the tightly packed coils in the compressed section of the slinky.
-
Rarefaction: This is the region where the particles are spread apart, resulting in a lower density. This is represented by the loosely spaced coils in the stretched section of the slinky after the compression wave has passed.
These alternating regions of compression and rarefaction constitute the wave itself. The distance between two consecutive compressions (or two consecutive rarefactions) defines the wavelength of the longitudinal wave.
Particle Displacement and Wave Velocity
It's crucial to differentiate between particle displacement and wave velocity.
-
Particle Displacement: This refers to the distance a single particle moves from its equilibrium position as the wave passes. This displacement is parallel to the wave's direction, oscillating back and forth around its resting point. The amplitude of the wave represents the maximum displacement of a particle from its equilibrium position.
-
Wave Velocity: This is the speed at which the wave itself propagates through the medium. This is independent of the individual particle's speed; the wave moves forward as energy is transmitted from particle to particle, even though individual particles only vibrate within a limited space.
Examples of Longitudinal Waves in Everyday Life
Longitudinal waves are prevalent in our daily experiences:
Sound Waves: The quintessential example
Sound waves are perhaps the most familiar example of longitudinal waves. Sound propagates through a medium (air, water, solids) as a series of compressions and rarefactions. When a sound source vibrates, it pushes the surrounding air molecules, creating compressions. These compressions then push adjacent molecules, creating a chain reaction that transmits the sound. The frequency of these compressions and rarefactions determines the pitch of the sound, while the amplitude determines the loudness.
Seismic P-waves: Earth's tremors
Seismic P-waves (primary waves) are longitudinal waves that travel through the Earth's interior following earthquakes. These waves are characterized by their relatively high speed and ability to travel through both solid and liquid mediums. The detection and analysis of P-waves are crucial in seismology for understanding the Earth's internal structure and locating earthquake epicenters.
Ultrasound: Medical applications
Ultrasound uses high-frequency longitudinal sound waves for medical imaging. These waves are transmitted into the body, and the echoes reflected from different tissues are used to create images. The differing densities of tissues affect the wave's propagation, enabling the visualization of internal organs and structures.
Spring oscillations: A visual demonstration
A simple spring provides an excellent visual representation of longitudinal waves. When a spring is compressed or stretched and then released, a longitudinal wave propagates along its length. The coils move back and forth along the spring's axis, demonstrating compression and rarefaction.
Mathematical Description of Longitudinal Waves
The behavior of longitudinal waves can be described mathematically using wave equations. These equations relate wave parameters such as wavelength (λ), frequency (f), and velocity (v) through the fundamental relationship:
v = fλ
where:
- v represents the wave velocity
- f represents the frequency of the wave
- λ represents the wavelength of the wave
More complex equations describe the displacement of individual particles within the wave as a function of time and position. These often involve sinusoidal functions that capture the oscillatory nature of the particle motion.
The Difference Between Longitudinal and Transverse Waves
It's essential to contrast longitudinal waves with transverse waves. In transverse waves, the particle oscillation is perpendicular to the direction of energy propagation. Examples include:
- Light waves: Electromagnetic waves, including light, are transverse waves. The electric and magnetic fields oscillate perpendicular to the direction of wave travel.
- Water waves: The motion of water particles in a surface wave is primarily circular or elliptical, with components both parallel and perpendicular to the direction of wave propagation. However, the energy transfer is predominantly along the water's surface.
- Waves on a string: When you pluck a guitar string, the string vibrates up and down (perpendicular to the string's length), creating a transverse wave.
The key difference lies in the direction of particle oscillation relative to wave propagation. This difference leads to distinct properties and behaviors for each type of wave.
Factors Affecting Wave Speed in Longitudinal Waves
The speed of a longitudinal wave depends on the properties of the medium through which it travels. Key factors include:
-
Density of the medium: Waves generally travel faster in denser media. This is because denser materials offer stronger intermolecular forces, enabling quicker transfer of energy between particles.
-
Elasticity (or stiffness) of the medium: Stiffer materials transmit longitudinal waves more efficiently. Stiffness relates to how readily a material resists deformation; higher stiffness leads to faster wave propagation.
-
Temperature: In many cases, temperature influences both density and elasticity, thus affecting wave speed. For instance, the speed of sound in air increases with temperature.
Applications of Longitudinal Waves
Longitudinal waves have a wide range of applications in various fields:
-
Medical Imaging (Ultrasound): As previously mentioned, ultrasound uses longitudinal waves for non-invasive imaging of internal organs and tissues.
-
Non-destructive testing: Longitudinal waves are employed to detect flaws and cracks in materials without causing damage. The reflection patterns of the waves provide information about internal structures.
-
Geophysics (Seismic Exploration): Longitudinal waves are crucial in geophysical surveys to explore subsurface structures and identify resources like oil and gas.
-
Sonar (Sound Navigation and Ranging): Sonar utilizes sound waves (longitudinal waves) to detect and locate objects underwater.
-
Acoustic Engineering: Understanding and controlling sound waves is critical in architectural acoustics, music production, and noise reduction.
Advanced Concepts and Further Exploration
For those interested in deeper exploration, the following concepts warrant further investigation:
-
Wave interference: The interaction of two or more longitudinal waves, resulting in constructive or destructive interference patterns.
-
Wave superposition: The principle that the net displacement of a particle in a medium is the sum of the displacements caused by individual waves passing through that point.
-
Wave diffraction: The bending of waves around obstacles or through narrow openings.
-
Wave refraction: The change in direction of waves when they pass from one medium to another.
-
Doppler effect: The apparent change in frequency of a wave due to the relative motion between the source and the observer.
Understanding longitudinal waves is crucial in various scientific disciplines and engineering applications. Their unique properties, described by the parallel motion of particles, make them a vital component of our physical world, with applications ranging from medical imaging to geological exploration. By grasping the fundamental principles outlined in this article, you can gain a comprehensive understanding of these fascinating waves and their significant roles in our world.
Latest Posts
Latest Posts
-
How To Measure The Wavelength Of A Transverse Wave
Mar 30, 2025
-
70 Is What Percent Of 80
Mar 30, 2025
-
How Many Feet Is 236 Inches
Mar 30, 2025
-
Least Common Multiple Of 10 And 5
Mar 30, 2025
-
Difference Between Chemical Change And Chemical Property
Mar 30, 2025
Related Post
Thank you for visiting our website which covers about In A Longitudinal Wave The Particles Move . We hope the information provided has been useful to you. Feel free to contact us if you have any questions or need further assistance. See you next time and don't miss to bookmark.