The Splitting Apart Of Atomic Nuclei Is Known As .
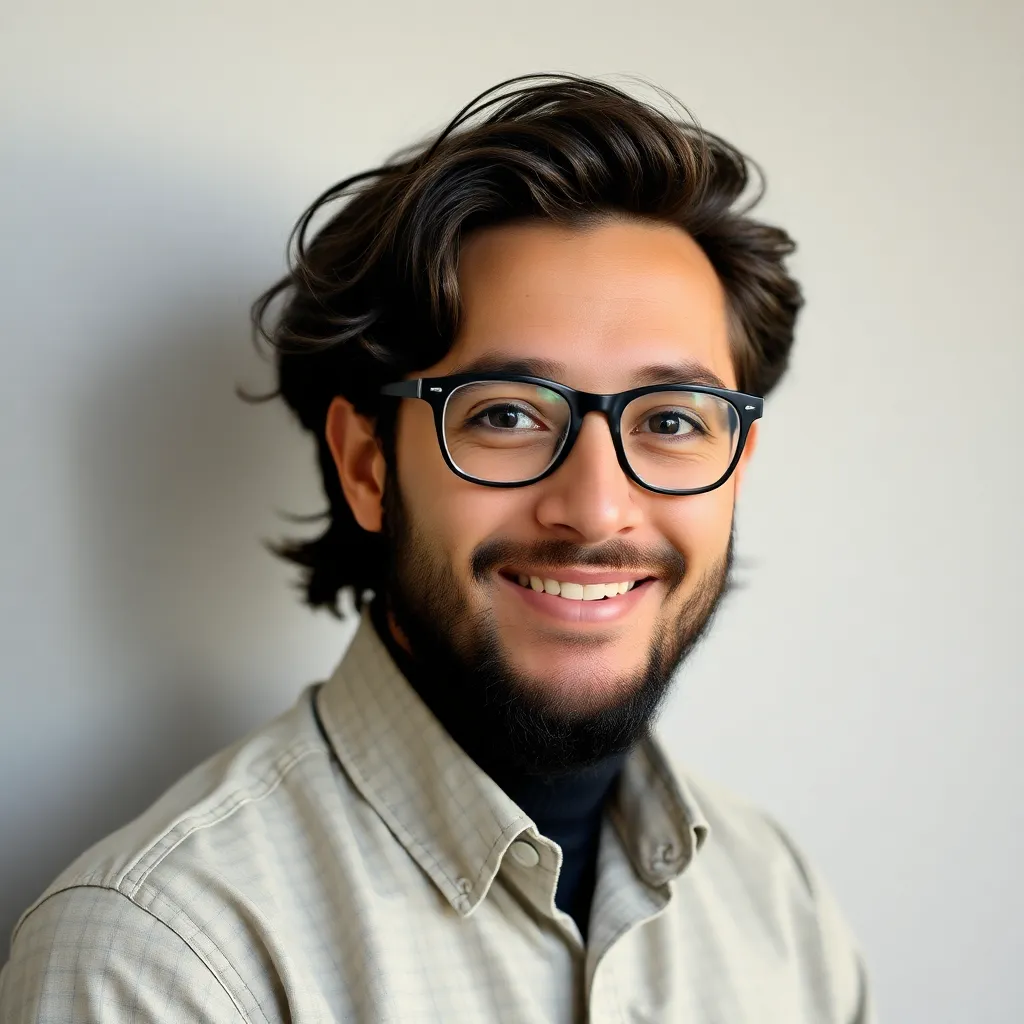
Kalali
Apr 15, 2025 · 6 min read
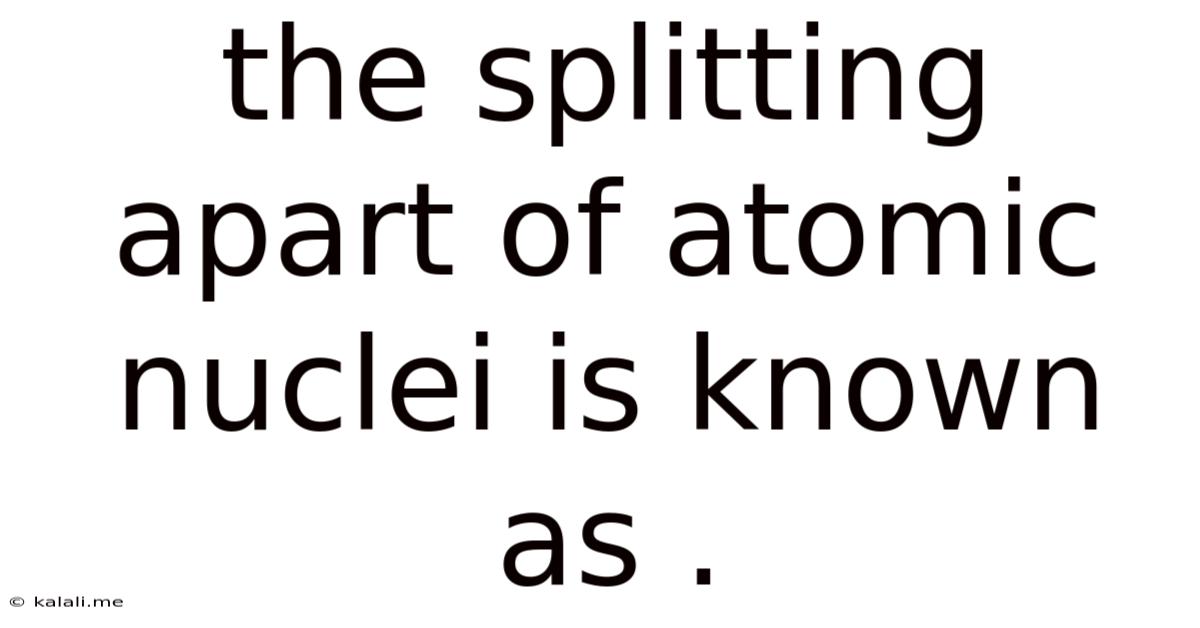
Table of Contents
The Splitting Apart of Atomic Nuclei is Known as Nuclear Fission: A Deep Dive into the Process, Applications, and Implications
The splitting apart of atomic nuclei is known as nuclear fission. This process, discovered in the late 1930s, has profoundly impacted our world, shaping everything from energy production to medical advancements and, unfortunately, the development of devastating weapons. Understanding nuclear fission requires delving into the intricacies of atomic structure, the forces governing subatomic particles, and the chain reactions that can be unleashed. This article explores these aspects, examining the mechanics of fission, its various applications, and the associated safety concerns and environmental impacts.
What is Nuclear Fission?
At its core, nuclear fission is the process where a heavy atomic nucleus, typically uranium-235 or plutonium-239, splits into two or more lighter nuclei. This splitting releases an immense amount of energy, along with free neutrons. These neutrons can then trigger further fission events in nearby nuclei, creating a self-sustaining chain reaction. The energy released manifests as kinetic energy of the fission fragments and as gamma radiation. This energy release is far greater than that observed in chemical reactions, making nuclear fission a potent source of power.
The process is initiated by bombarding the heavy nucleus with a neutron. This neutron absorption destabilizes the nucleus, causing it to become highly unstable and ultimately split. The resulting lighter nuclei, known as fission products, are often radioactive isotopes, meaning they undergo further radioactive decay, emitting particles and energy over time. The exact fission products vary, depending on the parent nucleus and the energy of the incident neutron.
The Mechanics of Nuclear Fission: A Closer Look
Several key factors govern the process of nuclear fission:
-
Nuclear Binding Energy: The stability of a nucleus is determined by its binding energy – the energy required to disassemble the nucleus into its constituent protons and neutrons. Heavy nuclei have relatively lower binding energy per nucleon compared to nuclei of intermediate mass. This means that splitting a heavy nucleus into lighter ones releases energy, as the resulting nuclei have higher binding energy per nucleon. This energy difference is the source of the massive energy released in fission.
-
Neutron Absorption: The initiation of fission depends on the absorption of a neutron by the heavy nucleus. The specific isotopes of uranium and plutonium used in fission reactors are carefully chosen for their high probability of neutron absorption. The absorbed neutron adds to the nucleus's mass and destabilizes it, triggering the fission process.
-
Fission Fragments: The splitting of the nucleus isn't perfectly symmetrical. The resulting fission fragments usually have different masses, ranging from roughly 70 to 160 atomic mass units. These fragments are highly energetic and radioactive, requiring careful handling and containment.
-
Neutron Emission: The fission process also releases several free neutrons (typically 2-3 per fission event). These neutrons are crucial for sustaining a chain reaction. If a sufficient number of these neutrons are absorbed by other fissile nuclei, a self-sustaining chain reaction is established. This is the principle behind nuclear reactors and atomic bombs.
-
Critical Mass: The minimum amount of fissile material required to sustain a chain reaction is known as the critical mass. If the amount of fissile material is below the critical mass, most of the neutrons escape without causing further fission, and the reaction dies out. Above the critical mass, the chain reaction grows exponentially, leading to a rapid release of energy.
Types of Nuclear Fission:
While the basic principle remains the same, variations exist in how fission is induced and controlled:
-
Spontaneous Fission: Some heavy nuclei can undergo fission spontaneously, without external neutron bombardment. This occurs rarely but contributes to the background radiation level.
-
Induced Fission: This is the most common type, triggered by the absorption of a neutron. The energy of the incident neutron influences the likelihood and outcome of fission.
-
Thermal Neutron Fission: Fission induced by low-energy (thermal) neutrons is crucial for controlled nuclear reactors. Moderators, such as graphite or water, are used to slow down fast neutrons produced during fission, increasing the probability of thermal neutron absorption.
-
Fast Neutron Fission: Some isotopes, such as plutonium-239, can undergo fission even with high-energy (fast) neutrons. This is relevant in fast breeder reactors and certain types of nuclear weapons.
Applications of Nuclear Fission:
The immense energy released during nuclear fission has several significant applications:
-
Nuclear Power Generation: Nuclear power plants utilize controlled chain reactions to generate electricity. The heat produced by fission is used to boil water, creating steam that drives turbines connected to generators. This is a low-carbon source of energy, although it does produce radioactive waste.
-
Nuclear Medicine: Radioisotopes produced through fission are used in various medical applications, including diagnosis (e.g., medical imaging) and treatment (e.g., radiation therapy). These isotopes emit radiation that can be detected or used to target cancerous cells.
-
Nuclear Weapons: Unfortunately, the destructive potential of uncontrolled nuclear fission led to the development of atomic bombs. The rapid, uncontrolled chain reaction in these weapons releases devastating amounts of energy, causing widespread destruction and long-term radioactive contamination.
-
Research and Scientific Applications: Fission research continues to contribute to our understanding of nuclear physics, material science, and related fields. Research reactors are used for various scientific purposes, including material testing and isotope production.
Safety Concerns and Environmental Impacts:
The use of nuclear fission involves significant safety concerns and environmental impacts:
-
Nuclear Waste: Nuclear fission produces radioactive waste, which remains hazardous for thousands of years. Safe and effective long-term storage of this waste is a major challenge.
-
Nuclear Accidents: Although rare, accidents in nuclear power plants can have devastating consequences, releasing large amounts of radioactivity into the environment (e.g., Chernobyl, Fukushima).
-
Nuclear Proliferation: The potential for misuse of fissile material for weapons production poses a significant global security threat. International efforts are focused on preventing the proliferation of nuclear weapons.
-
Radiation Hazards: Exposure to ionizing radiation from nuclear fission can cause various health problems, including cancer and genetic mutations. Strict safety measures are necessary to protect workers and the public from radiation exposure.
Future of Nuclear Fission:
Despite the challenges, nuclear fission remains a significant energy source, and ongoing research focuses on improving safety and sustainability:
-
Gen IV Reactors: Next-generation nuclear reactors are designed to improve safety, efficiency, and waste management. These reactors aim to reduce the volume and radioactivity of nuclear waste, enhance safety features, and potentially utilize nuclear fuel more efficiently.
-
Thorium Reactors: Thorium is a more abundant and less radioactive alternative to uranium. Research is ongoing to develop thorium-based reactors that offer enhanced safety and waste reduction potential.
-
Nuclear Fusion: While not directly related to fission, nuclear fusion is being explored as a cleaner and potentially safer alternative energy source. Fusion reactions produce significantly less radioactive waste than fission.
In conclusion, the splitting apart of atomic nuclei, nuclear fission, is a powerful process with far-reaching implications. Its applications in energy production and medicine have greatly benefited humanity, but the associated risks, including the production of radioactive waste and the potential for weapons proliferation, must be carefully managed. Ongoing research and development efforts aim to harness the benefits of nuclear fission while mitigating its risks, paving the way for safer and more sustainable energy solutions in the future. The quest for a cleaner and more efficient energy future continues, and the understanding and responsible application of nuclear fission will play a vital role in shaping that future. Further research into advanced reactor designs and alternative fuels like thorium offers a promising pathway towards safer and more sustainable nuclear energy. The continued development and deployment of robust safety protocols and efficient waste management strategies are crucial for ensuring the responsible use of this powerful technology.
Latest Posts
Latest Posts
-
How Many Mls In 14 Ounces
Apr 16, 2025
-
How Long Is 100mm In Inches
Apr 16, 2025
-
What Is 5 Out Of 7 As A Percentage
Apr 16, 2025
-
Is Bleaching Your Hair A Chemical Change
Apr 16, 2025
-
24 Is What Percent Of 80
Apr 16, 2025
Related Post
Thank you for visiting our website which covers about The Splitting Apart Of Atomic Nuclei Is Known As . . We hope the information provided has been useful to you. Feel free to contact us if you have any questions or need further assistance. See you next time and don't miss to bookmark.