What Is A Horizontal Row On The Periodic Table Called
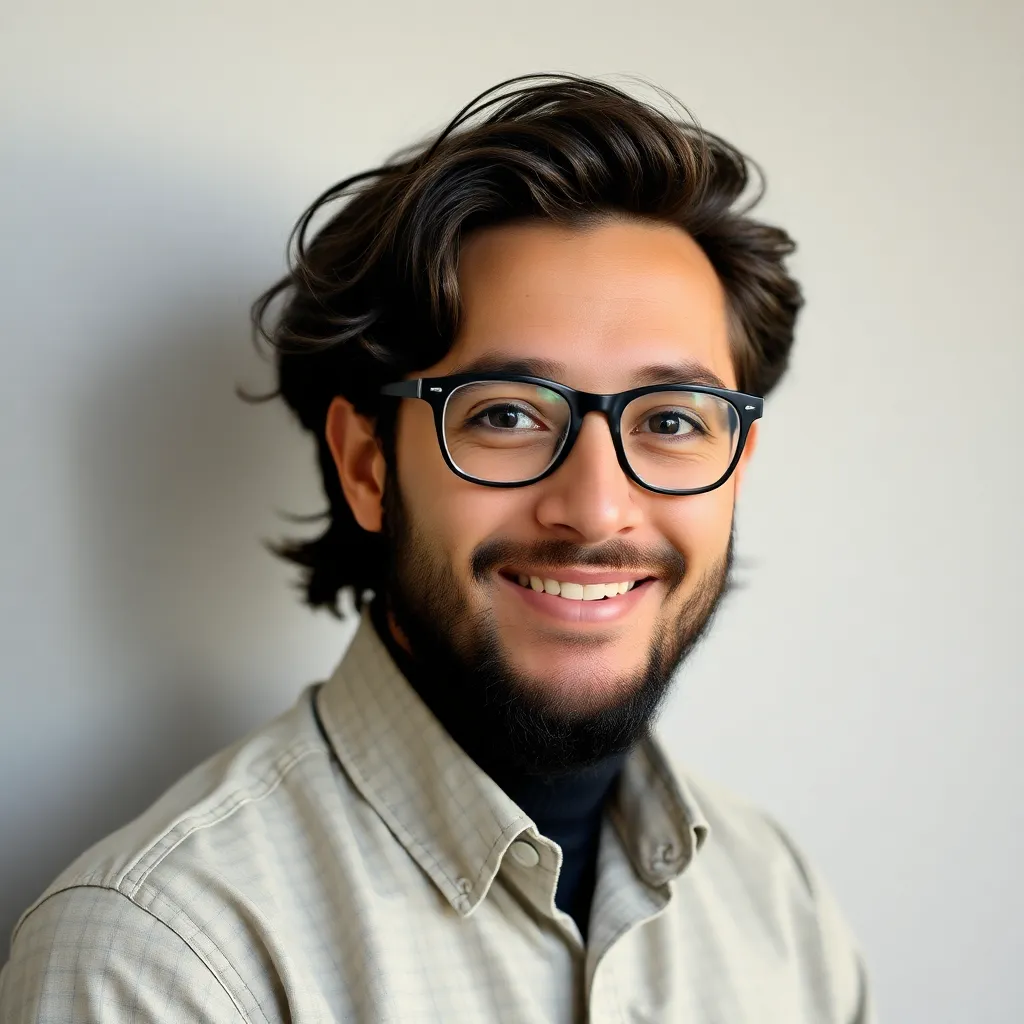
Kalali
Mar 08, 2025 · 7 min read
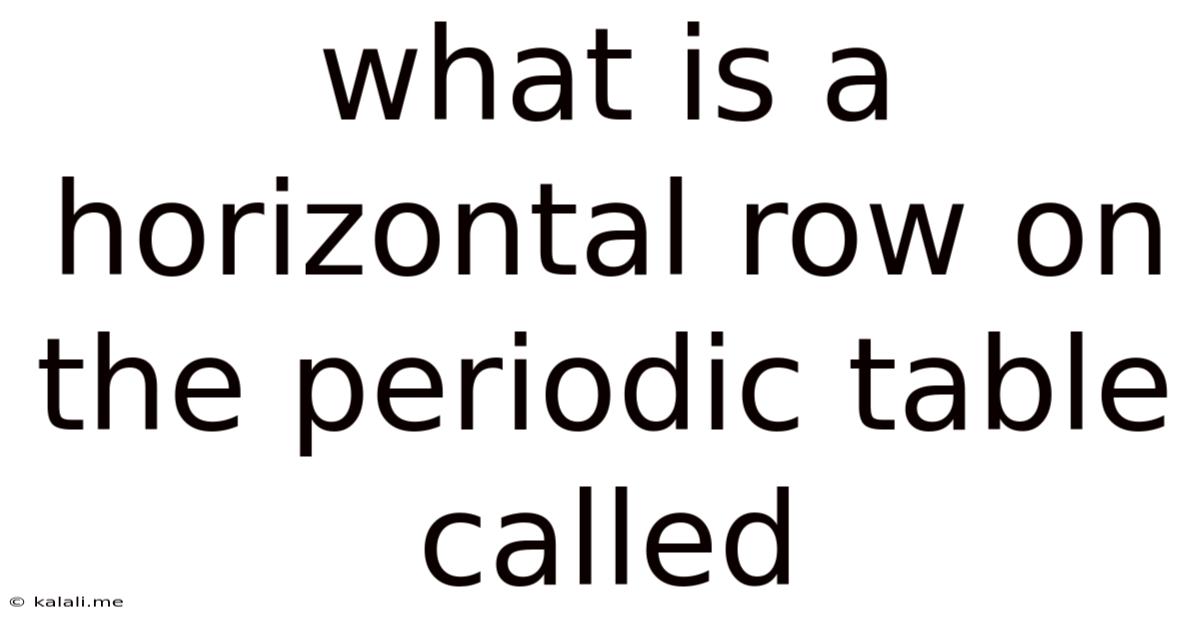
Table of Contents
What is a Horizontal Row on the Periodic Table Called? A Deep Dive into Periods and Their Significance
The periodic table, a cornerstone of chemistry, organizes elements based on their atomic number and recurring chemical properties. Understanding its structure is crucial for comprehending chemical behavior and predicting reactions. A common question among students and enthusiasts alike is: what is a horizontal row on the periodic table called? The answer is simple: a period. But understanding the significance of periods goes far beyond a simple definition. This article will explore periods in detail, examining their characteristics, trends, and the underlying reasons for their arrangement.
Understanding Periods: A Horizontal Journey Through Atomic Structure
Periods are the horizontal rows in the periodic table. Each period corresponds to a principal energy level (shell) in an atom's electron configuration. As we move across a period from left to right, the number of electrons in the outermost shell (valence shell) increases incrementally. This increase in valence electrons directly influences the chemical properties of the elements within that period.
Period 1: The Simplest of Beginnings
Period 1, the shortest period, contains only two elements: hydrogen (H) and helium (He). These elements fill the first principal energy level, which can accommodate a maximum of two electrons. Hydrogen, with one electron, is highly reactive, readily forming bonds with other elements. Helium, with a full valence shell (two electrons), is an inert noble gas, displaying remarkable chemical stability.
Period 2 and 3: The Rise of Blocks
Periods 2 and 3 are longer and introduce the concept of blocks within the periodic table. The elements in these periods are divided into s-block and p-block elements. The s-block elements (Groups 1 and 2) are characterized by their valence electrons in the s orbital, while the p-block elements (Groups 13-18) fill their p orbitals. This variation in electron configuration leads to a broader range of chemical properties within these periods compared to Period 1.
For example, Period 2 includes elements like lithium (Li), a reactive alkali metal, beryllium (Be), an alkaline earth metal, boron (B), a metalloid, carbon (C), a nonmetal forming the basis of organic chemistry, nitrogen (N), a crucial component of proteins and nucleic acids, oxygen (O), essential for respiration, fluorine (F), the most reactive nonmetal, and neon (Ne), an inert noble gas. This diversity highlights the impact of increased electron numbers and orbital filling on element behavior.
Period 4 and Beyond: The Introduction of d-Block Elements
Periods 4 and beyond introduce a new complexity: the d-block elements (transition metals). The filling of the d orbitals adds another layer to the electron configuration, resulting in a wider variety of oxidation states and complex ion formation. These transition metals are known for their catalytic activity, vibrant colors, and magnetic properties.
The inclusion of the d-block elements significantly lengthens these periods. The subsequent periods (5, 6, and 7) also include the f-block elements (lanthanides and actinides), which fill the f orbitals and are placed separately at the bottom of the table to maintain the table's manageable width. These elements are characterized by similar chemical properties within their respective series (lanthanides and actinides).
Periodic Trends within Periods: A Systematic Variation
As we traverse a period from left to right, several crucial periodic trends emerge, reflecting the systematic increase in effective nuclear charge and changes in atomic radius and electronegativity.
Atomic Radius: A Shrinking Trend
Atomic radius generally decreases across a period. This is because, while the number of electrons increases, the positive charge of the nucleus also increases. The stronger nuclear attraction pulls the electrons closer to the nucleus, resulting in a smaller atomic radius. The added electrons are added to the same principal energy level, experiencing similar shielding effects, therefore the increase in nuclear charge dominates the trend.
Ionization Energy: The Energy of Electron Removal
Ionization energy, the energy required to remove an electron from an atom, generally increases across a period. This is a direct consequence of the increased nuclear charge. The stronger attraction between the nucleus and electrons makes it increasingly difficult to remove an electron. Exceptions exist, particularly when a new subshell begins to fill, due to electron-electron repulsions.
Electronegativity: Attraction for Electrons
Electronegativity, the ability of an atom to attract electrons in a chemical bond, also generally increases across a period. This is because of the increase in effective nuclear charge, which enhances the atom's pull on shared electrons. The most electronegative elements are found in the upper right corner of the periodic table, excluding the noble gases.
Electron Affinity: Gaining Electrons
Electron affinity, the energy change associated with adding an electron to an atom, generally increases across a period. However, this trend is less regular than ionization energy and electronegativity due to the complexities of electron-electron repulsions in the already occupied orbitals.
The Importance of Periods in Predicting Chemical Behavior
Understanding the periodic trends within periods is critical for predicting the chemical behavior of elements. For example, the increasing electronegativity across a period explains why elements on the right side of the periodic table tend to gain electrons in chemical reactions (forming anions), while elements on the left tend to lose electrons (forming cations). This knowledge is fundamental for predicting the type of bonds formed (ionic, covalent, metallic) and the properties of the resulting compounds.
Furthermore, the periodic table's arrangement allows us to predict the reactivity of elements. Highly reactive metals (alkali metals) are found in Group 1, while highly reactive nonmetals (halogens) are found in Group 17. The noble gases in Group 18, with their full valence shells, exhibit exceptional inertness.
Periods and Chemical Reactions: A Practical Application
The periodic table's structure, especially the organization into periods, is essential for understanding and predicting the outcomes of chemical reactions. By examining the position of elements within a period, chemists can anticipate the types of bonds they will form, the reactivity they will display, and the properties of the resulting compounds.
For example, the reaction between an alkali metal (Group 1) and a halogen (Group 17) is highly predictable. The alkali metal readily loses one electron to achieve a stable electron configuration, while the halogen readily gains one electron. The electrostatic attraction between the resulting cation and anion forms an ionic compound. This simple prediction is a direct consequence of the arrangement of elements within the periods and their electron configurations.
Similarly, the reactivity of elements within a period can be used to predict the outcome of reactions involving more complex compounds. For instance, the relative electronegativities of elements within a period can be used to predict the direction of electron flow in a chemical reaction, determining which species will act as an oxidant or reductant.
Beyond the Basics: Deeper Insights into Periodicity
The concept of periods extends beyond simple trends in atomic properties. The periodicity of properties is also reflected in various other aspects of chemistry, including:
-
Spectroscopy: The electronic transitions within atoms, responsible for absorption and emission spectra, exhibit periodic patterns reflecting the electron configurations within periods.
-
Crystallography: The crystal structures of elements and compounds often show periodic relationships linked to atomic size and bonding characteristics determined by their position within a period.
-
Catalysis: The catalytic activity of transition metals, predominantly found in the d-block within certain periods, is a crucial aspect of many industrial processes. Their variable oxidation states and coordination complexes directly correlate with their placement in the periodic table.
Conclusion: Periods as the Foundation of Chemical Understanding
The horizontal rows of the periodic table, known as periods, are fundamental to understanding the organization and behavior of elements. The progressive filling of electron shells across a period leads to systematic variations in atomic size, ionization energy, electronegativity, and other properties. These trends are essential for predicting chemical reactions and understanding the properties of elements and their compounds. Mastering the concept of periods is crucial for any aspiring chemist or anyone seeking a deeper understanding of the fascinating world of chemical elements and their interactions. From the simplest reactions to the most complex catalytic processes, the principles governing periods provide a powerful framework for understanding and predicting chemical phenomena. The systematic nature of periods within the periodic table underscores its value as a cornerstone of chemical knowledge and a powerful tool for scientific discovery.
Latest Posts
Latest Posts
-
How Many Eighths In A Quarter Pound
Jul 18, 2025
-
Can The Sine Of An Angle Ever Equal 2
Jul 18, 2025
-
How Many Months Is A Hundred Days
Jul 18, 2025
-
Mother And I Or Mother And Me
Jul 18, 2025
-
How Many Oz In One Water Bottle
Jul 18, 2025
Related Post
Thank you for visiting our website which covers about What Is A Horizontal Row On The Periodic Table Called . We hope the information provided has been useful to you. Feel free to contact us if you have any questions or need further assistance. See you next time and don't miss to bookmark.