What Is The Physical Basis Of The Phototropic Response
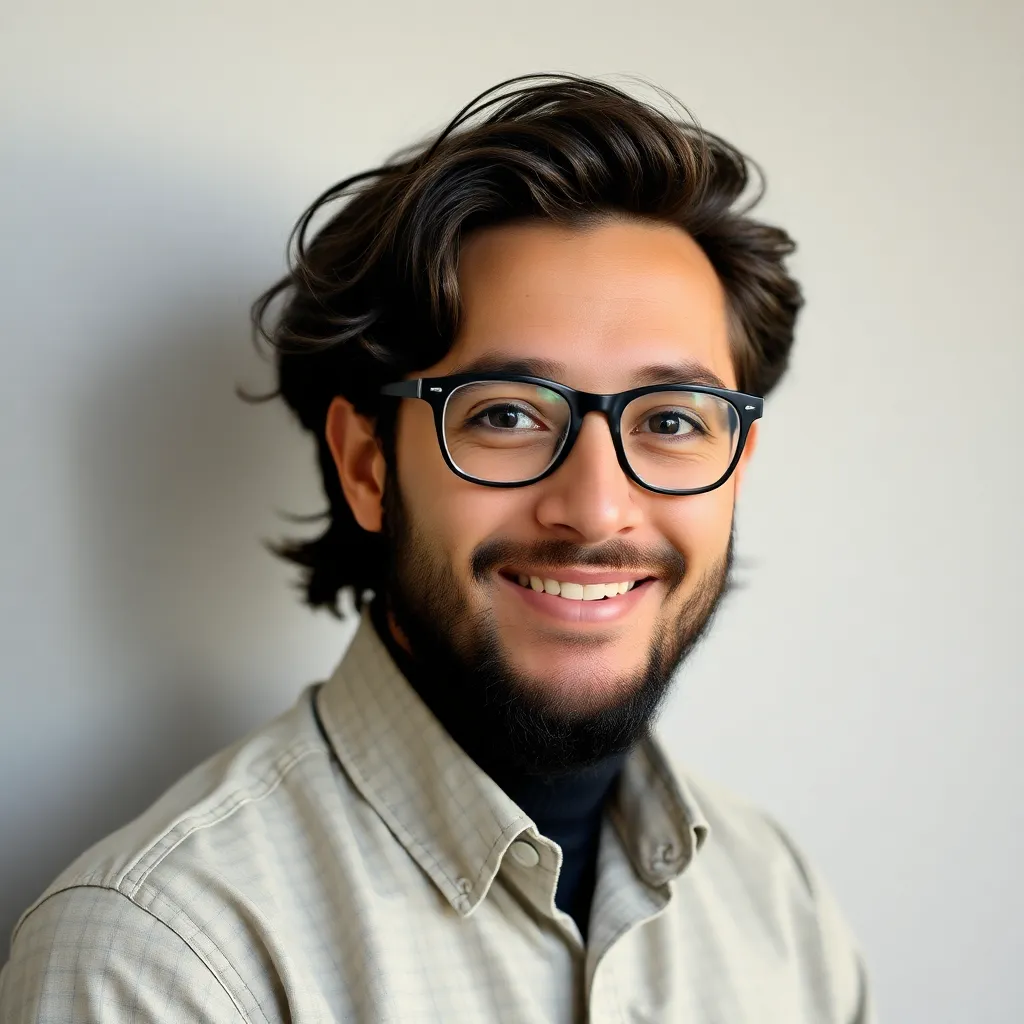
Kalali
Mar 30, 2025 · 7 min read
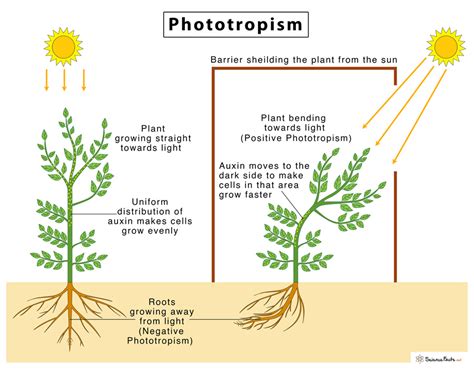
Table of Contents
What is the Physical Basis of the Phototropic Response?
Plants, those silent sentinels of our world, possess an incredible ability to sense and respond to their environment. One of the most striking examples of this is phototropism, the directional growth of a plant in response to a light source. This seemingly simple bending towards light is, in fact, a complex interplay of intricate physical and chemical processes. Understanding the physical basis of this response reveals a fascinating tale of cellular mechanics, signaling pathways, and the elegant adaptation of plants to their surroundings.
The Role of Light Perception: Photoreceptors and Signaling
The journey begins with the perception of light itself. Plants, unlike animals with their sophisticated eyes, employ specialized photoreceptors – proteins that absorb light and trigger a downstream cascade of events. The key player in phototropism is phototropin, a flavoprotein that absorbs blue light most effectively. This sensitivity to blue light makes perfect evolutionary sense, as blue light is the most abundant component of sunlight in the photosynthetically active range.
Phototropin Activation: A Molecular Switch
When a phototropin molecule absorbs blue light, it undergoes a conformational change, essentially flipping a molecular switch. This change activates the phototropin's kinase activity, enabling it to phosphorylate other proteins. This phosphorylation event initiates a complex signaling cascade, involving numerous second messengers and intermediary proteins. The exact details of this cascade are still being actively researched, but it ultimately leads to changes in the plant's growth pattern.
Auxin: The Master Regulator of Phototropism
At the heart of the phototropic response lies auxin, a plant hormone crucial for a wide range of developmental processes. In the context of phototropism, auxin acts as the primary signal transducer, orchestrating the differential growth that leads to bending. Upon light perception, auxin undergoes redistribution within the plant. This redistribution isn't a uniform process.
Lateral Auxin Redistribution: The Key to Bending
The crucial aspect of auxin's role is its lateral redistribution. When a plant is exposed to unilateral light (light from one side), auxin is transported from the illuminated side to the shaded side of the stem. This creates an auxin gradient, with higher concentrations on the shaded side and lower concentrations on the illuminated side. This gradient is the driving force behind the differential growth that results in bending towards the light source.
Cellular Mechanisms: Acid Growth Hypothesis and Cell Expansion
The increased auxin concentration on the shaded side doesn't directly cause growth. Instead, it triggers a series of cellular events that lead to differential cell elongation. The predominant model for this is the acid growth hypothesis.
Acid Growth Hypothesis: Proton Pumps and Cell Wall Loosening
According to the acid growth hypothesis, auxin stimulates the activity of proton pumps (H+-ATPases) located in the plasma membrane of cells. These pumps actively transport protons (H+) from the cytoplasm into the cell wall. This acidification of the cell wall reduces the pH, activating expansins, enzymes that loosen the cell wall's structure. This loosening allows the cells to expand more readily in response to turgor pressure, the pressure exerted by water within the cell.
Differential Cell Elongation: The Physical Bending
The combination of increased auxin, enhanced proton pumping, cell wall loosening, and turgor pressure creates a situation where cells on the shaded side elongate significantly faster than cells on the illuminated side. This differential elongation is the physical basis of the phototropic curvature. The shaded side grows longer, causing the stem to bend towards the light.
Beyond the Stem: Phototropism in Other Plant Organs
While the stem's phototropic response is perhaps the most readily observed, this phenomenon extends to other plant organs as well. Roots, for example, generally exhibit negative phototropism, growing away from the light. This difference in response stems from variations in the photoreceptors expressed and the auxin signaling pathways involved.
Root Phototropism: A Different Approach
In roots, the photoreceptor phytochrome plays a more prominent role than phototropin in mediating light perception. The signaling cascades triggered by phytochrome in roots are distinct from those in stems, leading to a different auxin distribution and resulting in negative phototropism. The exact mechanisms involved are still under investigation, but it’s clear that the root system has evolved sophisticated ways to avoid excessive light exposure.
Leaves and Other Organs: Light-Guided Development
Phototropism also affects leaf orientation and development. Leaves often position themselves optimally to capture light for photosynthesis. This involves a complex interplay of phototropism and other growth movements, ensuring maximum light interception. Similarly, other plant structures like tendrils and inflorescences also display varying degrees of phototropism, adapting their growth to optimize light capture and reproduction.
Factors Influencing Phototropism: Environmental and Genetic Considerations
The phototropic response isn't a static phenomenon; it's highly influenced by environmental and genetic factors. Light intensity, wavelength, duration of light exposure, and the presence of other environmental stimuli all affect the magnitude and direction of phototropism.
Light Intensity and Wavelength: Shaping the Response
Higher light intensities generally lead to a stronger phototropic response, as more photons are absorbed by photoreceptors. The wavelength of light is equally critical, with blue light being the most effective in triggering the response. Other wavelengths, like red and far-red light, can modulate the phototropic response but are less potent.
Duration of Light Exposure: Time Matters
The duration of light exposure also influences the magnitude of the phototropic response. Prolonged exposure to unilateral light typically results in greater curvature. This emphasizes the importance of both light perception and the sustained signaling cascade in achieving the final bending.
Genetic Variations: Diversity in Response
Genetic variations among different plant species and even within a species can significantly impact the phototropic response. Mutations in photoreceptors, auxin signaling components, or other genes involved in the process can alter the sensitivity and strength of phototropism. This genetic diversity is an evolutionary advantage, allowing plants to adapt to a wider range of light environments.
Future Directions: Unveiling the Complexity
Despite decades of research, the physical basis of the phototropic response remains a subject of active investigation. Researchers are still uncovering the intricacies of the auxin signaling cascade, the roles of other signaling molecules, and the precise mechanisms of cell wall expansion. Advanced techniques like genetic engineering, proteomics, and sophisticated microscopy are providing new insights into this fascinating area of plant biology.
Unraveling the Signaling Network: A Systems Biology Approach
Understanding the complete picture of phototropism necessitates a systems biology approach, integrating data from multiple levels, from individual genes and proteins to whole-plant responses. This requires advanced computational methods and interdisciplinary collaboration to unravel the intricate network of interactions that govern this fundamental process.
Beyond Auxin: Exploring Other Players
While auxin is central to phototropism, other plant hormones and signaling molecules likely play supporting roles. Identifying and characterizing these molecules will provide a more comprehensive understanding of the underlying mechanisms.
The Role of the Cytoskeleton: Guiding Growth
The cytoskeleton, the internal scaffolding of the cell, plays a critical role in many cellular processes, including cell growth and expansion. The involvement of the cytoskeleton in phototropism is still being investigated but promises to provide more insights into the mechanics of bending.
In conclusion, the physical basis of the phototropic response is a complex interplay of light perception, hormone signaling, and cellular mechanisms. While significant progress has been made in understanding the fundamental components of this process, many exciting avenues of research remain. The continuing investigation into phototropism not only enhances our understanding of plant biology but also has implications for agricultural practices and biotechnology, potentially leading to the development of crops with improved light-harvesting capabilities. The seemingly simple bending of a plant towards the light is, in fact, a marvel of biological engineering, a testament to the power of evolution and a fascinating subject of ongoing scientific inquiry.
Latest Posts
Latest Posts
-
Is Oxygen A Mixture Or Pure Substance
Apr 01, 2025
-
What Percentage Is 5 Out Of 7
Apr 01, 2025
-
Convert 16 Degrees Celsius To Fahrenheit
Apr 01, 2025
-
59 Cm Is How Many Inches
Apr 01, 2025
-
How Many Feet In 300 Meters
Apr 01, 2025
Related Post
Thank you for visiting our website which covers about What Is The Physical Basis Of The Phototropic Response . We hope the information provided has been useful to you. Feel free to contact us if you have any questions or need further assistance. See you next time and don't miss to bookmark.