Why Cellular Respiration Is Not Endergonic
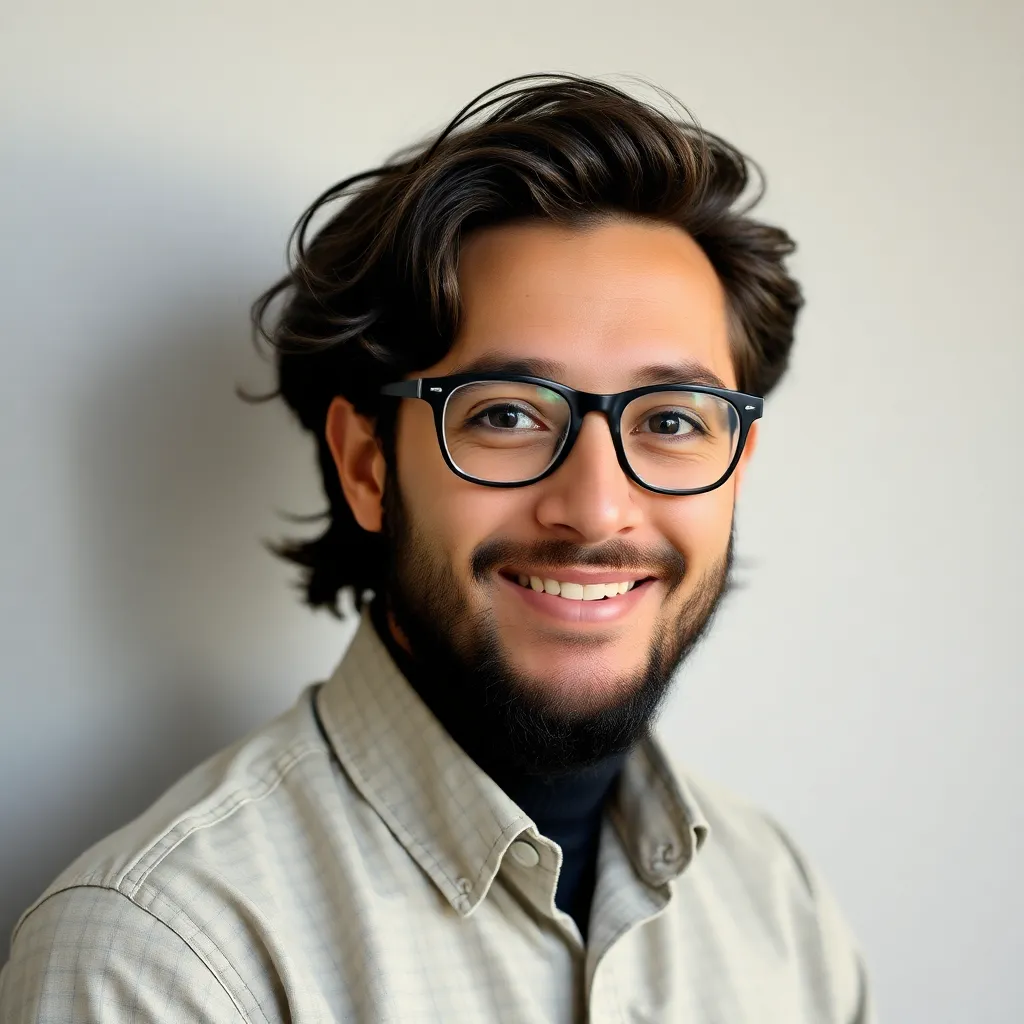
Kalali
Apr 17, 2025 · 6 min read
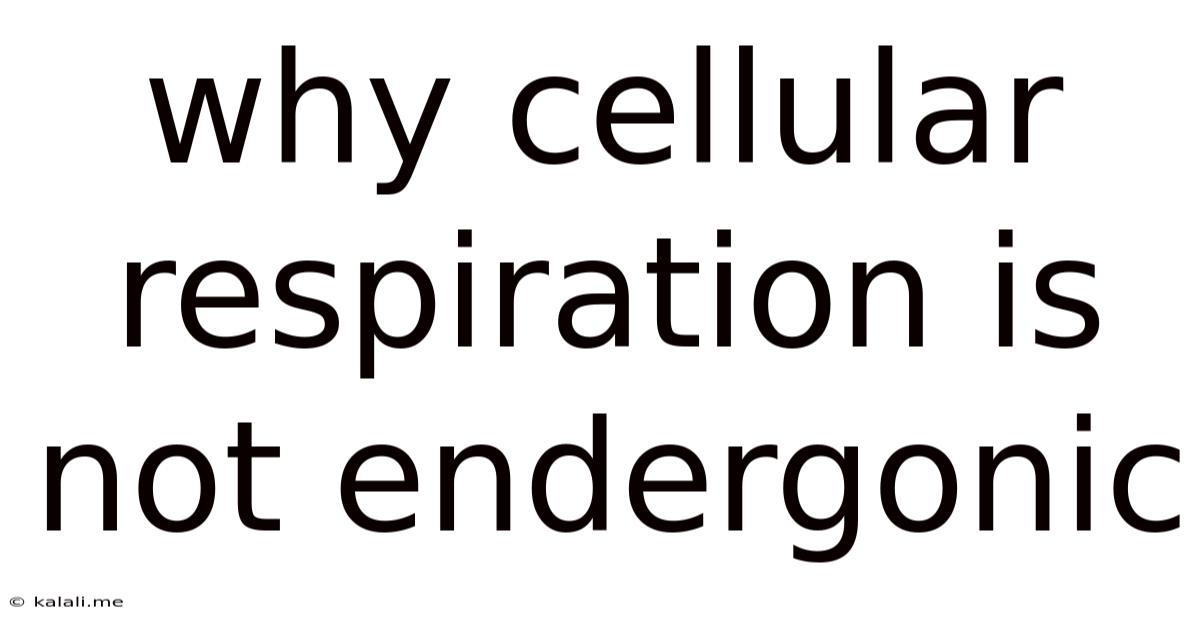
Table of Contents
Why Cellular Respiration Is Not Endergonic: A Deep Dive into Energy Production in Cells
Cellular respiration is a fundamental process in almost all living organisms, responsible for converting the chemical energy stored in glucose into a readily usable form of energy, ATP (adenosine triphosphate). A common misconception surrounds the energetic nature of this process. Many mistakenly believe cellular respiration to be endergonic, a process that requires energy input. However, this is incorrect. Cellular respiration is, in fact, exergonic, releasing energy in the process. This article will delve into the reasons why, exploring the thermodynamics of the process, the key steps involved, and the crucial role of electron carriers and the electron transport chain.
Cellular respiration can be summarized by the following overall equation: C₆H₁₂O₆ + 6O₂ → 6CO₂ + 6H₂O + ATP. This equation clearly demonstrates a release of energy, as the complex glucose molecule is broken down into simpler molecules (carbon dioxide and water). This energy release is the defining characteristic of an exergonic reaction. The negative Gibbs free energy change (ΔG < 0) associated with cellular respiration confirms its exergonic nature. Understanding this fundamental aspect is crucial for grasping the intricacies of cellular energy metabolism.
Understanding Endergonic vs. Exergonic Reactions
Before diving into the specifics of cellular respiration, it's crucial to understand the difference between endergonic and exergonic reactions. These terms describe the energy changes associated with chemical reactions.
-
Endergonic reactions require an input of energy to proceed. The products of an endergonic reaction have a higher free energy than the reactants. The ΔG for an endergonic reaction is positive (ΔG > 0). Examples include photosynthesis and the synthesis of complex molecules like proteins.
-
Exergonic reactions release energy as they proceed. The products of an exergonic reaction have a lower free energy than the reactants. The ΔG for an exergonic reaction is negative (ΔG < 0). Cellular respiration is a prime example, as are many catabolic processes involving the breakdown of molecules.
The Stages of Cellular Respiration: A Step-by-Step Exergonic Process
Cellular respiration is a multi-step process, broadly divided into four main stages: glycolysis, pyruvate oxidation, the Krebs cycle (also known as the citric acid cycle), and oxidative phosphorylation (including the electron transport chain and chemiosmosis). Each stage contributes to the overall exergonic nature of the process.
1. Glycolysis: Breaking Down Glucose
Glycolysis occurs in the cytoplasm and involves the breakdown of glucose (a six-carbon sugar) into two molecules of pyruvate (a three-carbon compound). While a small amount of ATP is invested at the beginning (making it seem initially endergonic), the net yield of ATP is significantly positive. This process generates a small amount of ATP through substrate-level phosphorylation and produces NADH, an electron carrier crucial for later stages. The release of energy during the conversion of glucose to pyruvate makes this stage fundamentally exergonic. The overall reaction is exergonic, with a negative ΔG.
2. Pyruvate Oxidation: Preparing for the Krebs Cycle
Pyruvate, produced during glycolysis, is transported into the mitochondria (in eukaryotes). Here, it undergoes oxidation, converting it into acetyl-CoA. This step releases carbon dioxide and generates NADH, further contributing to the exergonic nature of the process. The oxidation of pyruvate is an exergonic reaction, releasing energy that is captured in the form of NADH.
3. The Krebs Cycle (Citric Acid Cycle): Energy Harvesting in the Mitochondria
The Krebs cycle takes place in the mitochondrial matrix. Acetyl-CoA enters the cycle and undergoes a series of redox reactions, releasing carbon dioxide. This cycle generates a significant amount of NADH and FADH₂, electron carriers that will be critical for the electron transport chain. It also produces a small amount of ATP through substrate-level phosphorylation. The complete oxidation of acetyl-CoA is highly exergonic, contributing significantly to the overall energy yield of cellular respiration. Each turn of the cycle releases energy which is captured in the reducing power of NADH and FADH2 and a small amount of ATP.
4. Oxidative Phosphorylation: The Powerhouse of Cellular Respiration
Oxidative phosphorylation, occurring in the inner mitochondrial membrane (in eukaryotes), is the most significant energy-producing stage of cellular respiration. It involves two main processes:
-
Electron Transport Chain (ETC): Electrons from NADH and FADH₂, generated in the previous stages, are passed along a series of protein complexes embedded in the inner mitochondrial membrane. As electrons move down the chain, energy is released, used to pump protons (H⁺) from the mitochondrial matrix into the intermembrane space, creating a proton gradient. This process is highly exergonic, driven by the high reduction potential of oxygen (the final electron acceptor).
-
Chemiosmosis: The proton gradient generated by the ETC creates a potential energy difference across the inner mitochondrial membrane. Protons flow back into the matrix through ATP synthase, an enzyme that uses this proton motive force to synthesize ATP. This is called chemiosmosis, and it's a remarkable example of coupling an exergonic process (proton flow) to an endergonic process (ATP synthesis). However, the overall process remains exergonic, with the energy released from electron transport being the driving force. The massive ATP yield from this stage far outweighs the energy required for the initial proton pumping, making the entire process significantly exergonic.
The Role of Electron Carriers and the Electron Transport Chain
NADH and FADH₂ are crucial electron carriers. They accept electrons during the earlier stages of cellular respiration and deliver them to the electron transport chain. The electron transport chain is a series of redox reactions, where electrons are passed from one molecule to another, with a stepwise decrease in free energy. The highly electronegative oxygen acts as the final electron acceptor, forming water. This electron flow drives proton pumping, leading to ATP synthesis via chemiosmosis. The sequential transfer of electrons and the resulting proton gradient are essential components of the exergonic nature of oxidative phosphorylation.
Why the Misconception? Understanding the Sub-processes
The misconception that cellular respiration might be endergonic likely stems from focusing on individual steps rather than the whole process. While some individual steps within glycolysis require a small initial energy investment (phosphorylation), the overall energy balance is significantly positive, making glycolysis and the entire process exergonic. The energy released during these individual steps is stored in the high-energy phosphate bonds of ATP, or the reducing power of NADH and FADH2, which are then used to drive subsequent steps and contribute to the overall exergonic nature. The crucial point to remember is that the sum of the free energy changes of all the steps results in a negative overall ΔG.
Conclusion: Cellular Respiration – A Highly Efficient Exergonic Process
In conclusion, cellular respiration is demonstrably an exergonic process, releasing a large amount of energy captured in the form of ATP. The misconception arises from a lack of consideration of the entire process and focusing on individual steps that may appear endergonic in isolation. Understanding the four stages, the role of electron carriers, and the efficiency of the electron transport chain and chemiosmosis is crucial to appreciating the energetic nature of this essential metabolic pathway. The negative change in Gibbs free energy (ΔG < 0) associated with the overall process confirms its exergonic status and underpins its role as the primary energy-yielding pathway in most living organisms. The remarkable efficiency of cellular respiration in converting the energy stored in glucose into ATP is a testament to the elegance and precision of biological systems.
Latest Posts
Latest Posts
-
Cuanto Es 30 Pulgadas En Metros
Apr 19, 2025
-
Cuanto Es 50 Yardas En Metros
Apr 19, 2025
-
How Many Liters Is 70 Ounces
Apr 19, 2025
-
What Is The End Product Of Transcription
Apr 19, 2025
-
Cuanto Es 38 Centimetros En Pulgadas
Apr 19, 2025
Related Post
Thank you for visiting our website which covers about Why Cellular Respiration Is Not Endergonic . We hope the information provided has been useful to you. Feel free to contact us if you have any questions or need further assistance. See you next time and don't miss to bookmark.