What Drives Melting At Divergent Boundaries
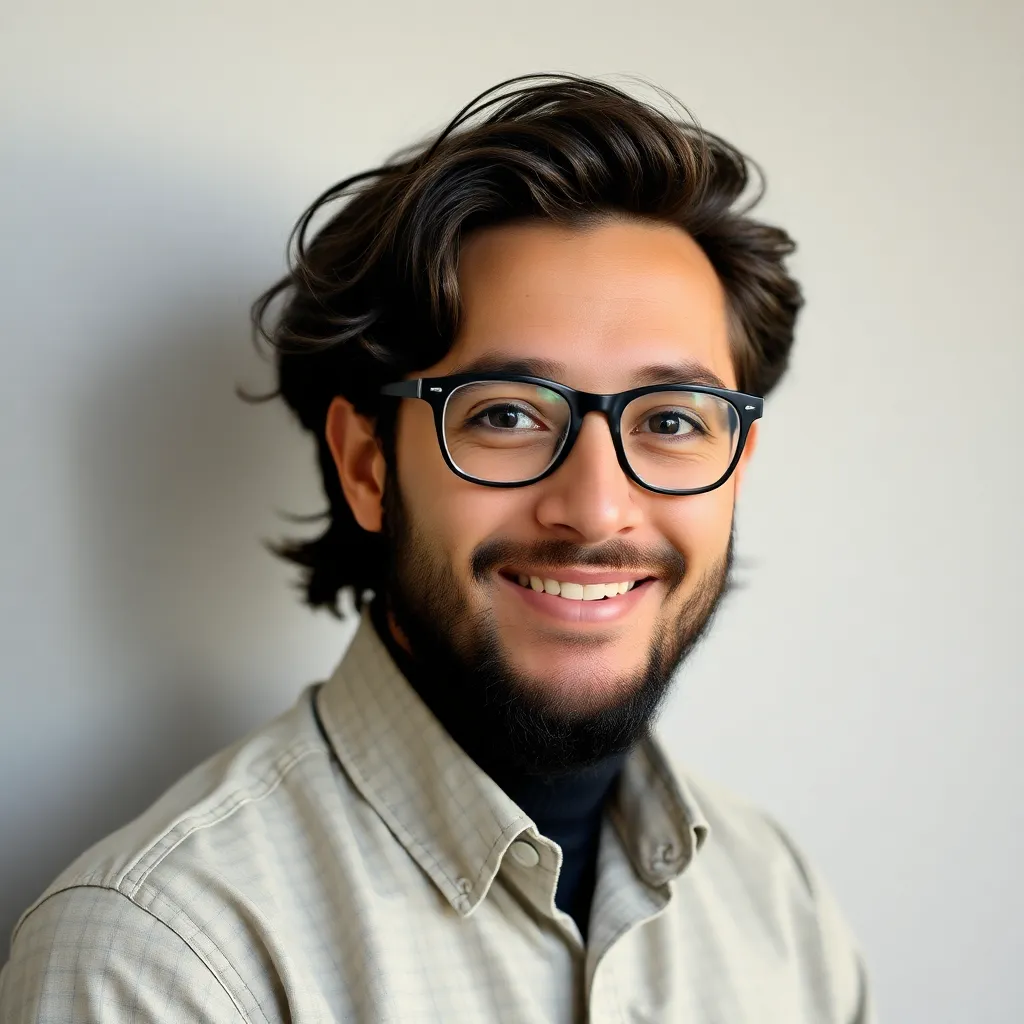
Kalali
Mar 29, 2025 · 6 min read
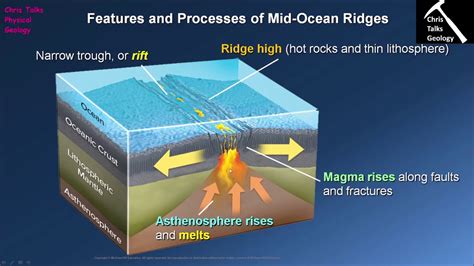
Table of Contents
What Drives Melting at Divergent Boundaries?
Divergent boundaries, where tectonic plates pull apart, are cradles of new oceanic crust. This creation is a direct consequence of magma generation and subsequent eruption at these plate margins. But what exactly drives this melting? It's not a simple answer, and understanding the process requires delving into the complex interplay of pressure, temperature, and composition within the Earth's mantle.
The Role of Decompression Melting
The primary mechanism driving melting at divergent boundaries is decompression melting. Imagine a sealed container of soda. When you open it, the pressure drops suddenly, and the dissolved carbon dioxide comes out of solution, forming bubbles. A similar process occurs in the Earth's mantle.
The Mantle Plume Model: A Simplified View
As tectonic plates move apart, they create space. This space is filled by the upwelling of hot mantle material from deeper regions. As this mantle material rises, it experiences a decrease in pressure. Crucially, the adiabatic ascent is relatively fast— meaning the heat doesn't have enough time to be lost to the surrounding rocks. This drop in pressure, without a significant decrease in temperature, lowers the melting point of the mantle rock. Once the pressure drops below the solidus (the temperature at which a material begins to melt), partial melting occurs.
Beyond the Simplified Model: The Complexity of Mantle Composition
The mantle isn't homogenous; it's a complex mixture of minerals with varying melting points. This compositional heterogeneity significantly influences the melting process. While decompression is the main driver, the presence of specific minerals, like those rich in water or carbon dioxide, can further lower the melting point and enhance the degree of melting.
Water's Significant Role: Even small amounts of water dissolved in the mantle can drastically reduce the melting point. This water, typically originating from subducted oceanic plates, can significantly increase the extent of melting at divergent boundaries, especially in mid-ocean ridges with high hydrothermal activity.
The Influence of Mantle Plumes: While decompression melting is the dominant factor, the interaction of mantle plumes with divergent boundaries can amplify the melting process. Mantle plumes are upwellings of exceptionally hot mantle material from deep within the Earth. Their interaction with spreading centers can lead to increased volcanism and higher rates of seafloor spreading, driven by elevated melting rates.
Other Contributing Factors: Temperature and Heat Transfer
While decompression is the dominant driver, temperature and heat transfer also play crucial roles.
Geothermal Gradient and Heat Flow: The Engine of Mantle Convection
The Earth's internal heat, primarily generated by radioactive decay in the mantle and core, drives mantle convection. This process, similar to boiling water in a pot, transfers heat from the Earth's interior towards the surface. The geothermal gradient, the rate at which temperature increases with depth, varies across the globe. Higher geothermal gradients in certain regions can increase the temperature of the upwelling mantle material, further contributing to melting.
The Importance of Heat Transfer Mechanisms: Conduction, Convection, and Advection
Heat transfer at divergent boundaries occurs through a combination of mechanisms:
- Conduction: Heat transfer through direct contact between molecules. While efficient within the solid mantle, conduction is relatively slow in transferring heat away from upwelling material.
- Convection: The movement of fluids (like the molten rock) carrying heat with them. This is a highly efficient heat transfer mechanism in the mantle, especially within the partially molten regions.
- Advection: The bulk transport of heat through the movement of the material itself. The rising mantle plumes demonstrate this effect effectively.
The Formation of Magma and Its Evolution
The partial melting generated by these processes doesn't create entirely molten rock immediately. Instead, it produces a mixture of molten rock (magma) and solid minerals. The proportion of melt generated depends on factors like the degree of decompression, initial temperature, mantle composition, and the presence of volatiles.
Magma Composition and Differentiation: A Story of Fractional Crystallization
The composition of the magma generated at divergent boundaries is largely determined by the composition of the source mantle rock. However, as the magma rises, it undergoes fractional crystallization, a process where minerals crystallize out of the melt at different temperatures. This changes the composition of the remaining liquid magma, making it richer in silica and other incompatible elements. This evolution significantly impacts the volcanic rocks formed at divergent boundaries.
The Role of Assimilation and Contamination
During its ascent, magma can interact with the surrounding rocks. A process called assimilation involves the incorporation of these surrounding rocks into the magma, altering its composition. This process can lead to variations in magma chemistry, producing diverse rock types at divergent boundaries.
Observing the Effects: Evidence from Mid-Ocean Ridges
Mid-ocean ridges, the most prominent example of divergent boundaries, provide compelling evidence for the melting processes discussed above.
Seismic Tomography: Imaging the Mantle's Interior
Seismic tomography uses seismic waves to create 3D images of the Earth's interior. This technique reveals low-velocity zones beneath mid-ocean ridges, indicating the presence of partially molten material. The extent and distribution of these zones provide valuable insights into the location and magnitude of melting.
Geothermal Flux and Heat Flow Measurements: Quantifying Heat Transfer
Measurements of geothermal flux (heat flow) at mid-ocean ridges show significantly higher values than average continental heat flow. This high heat flow directly reflects the upwelling of hot mantle material and the large amount of heat being transferred to the overlying oceanic crust.
Volcanic Activity and Seafloor Spreading: The Manifestation of Melting
The abundant volcanic activity along mid-ocean ridges is a direct consequence of magma generation and eruption. The rate of seafloor spreading, representing the rate at which new oceanic crust is formed, is closely linked to the rate of melting beneath the ridges. Faster spreading rates generally correlate with higher melting rates.
Variations in Melting and Their Global Implications
The processes described are not uniform across all divergent boundaries. Variations in spreading rate, mantle composition, and the presence of plumes lead to significant differences in the extent and style of magmatism.
Fast Spreading Ridges vs. Slow Spreading Ridges: A Contrast in Magmatism
Fast-spreading ridges, like the East Pacific Rise, generally have a greater volume of melt and a wider zone of partial melting than slow-spreading ridges, like the Mid-Atlantic Ridge. This difference is mainly due to the higher rates of decompression and mantle upwelling in fast-spreading systems.
The Influence of Mantle Plumes: Hotspot Volcanism at Divergent Boundaries
The interaction between mantle plumes and divergent boundaries can lead to significant anomalies in melting. Hotspot volcanism, characterized by elevated volcanic activity and distinct geochemical signatures, often occurs at or near divergent boundaries, providing strong evidence for the influence of mantle plumes on melting processes.
The Impact of Subduction Zones: The Water Puzzle
Subduction zones, where oceanic plates sink beneath continental plates, are sources of water that are transported into the mantle wedge. This influx of water can significantly influence melting processes at nearby divergent boundaries, especially those located close to subduction zones.
Conclusion: A Dynamic System Driven by Multiple Factors
The melting at divergent boundaries is a dynamic process governed by the interplay of decompression melting, temperature, mantle composition, and heat transfer. While decompression melting is the primary driver, the influence of mantle plumes, water content, and variations in spreading rates creates a diverse range of magmatic environments. Understanding these complex interactions is crucial for comprehending the formation of oceanic crust, the evolution of plate tectonics, and the global distribution of heat and mass within the Earth's interior. Continued research using advanced geophysical techniques and geochemical analyses will further refine our understanding of these vital geological processes.
Latest Posts
Latest Posts
-
How Much Is 70 Ounces Of Water
Apr 01, 2025
-
Cuanto Es 56 Grados Fahrenheit En Centigrados
Apr 01, 2025
-
115 Out Of 125 As A Percentage
Apr 01, 2025
-
What Is 10 5 Inches In Cm
Apr 01, 2025
-
How To Find X And Y Intercepts In Vertex Form
Apr 01, 2025
Related Post
Thank you for visiting our website which covers about What Drives Melting At Divergent Boundaries . We hope the information provided has been useful to you. Feel free to contact us if you have any questions or need further assistance. See you next time and don't miss to bookmark.