What Is Found In Rna But Not Dna
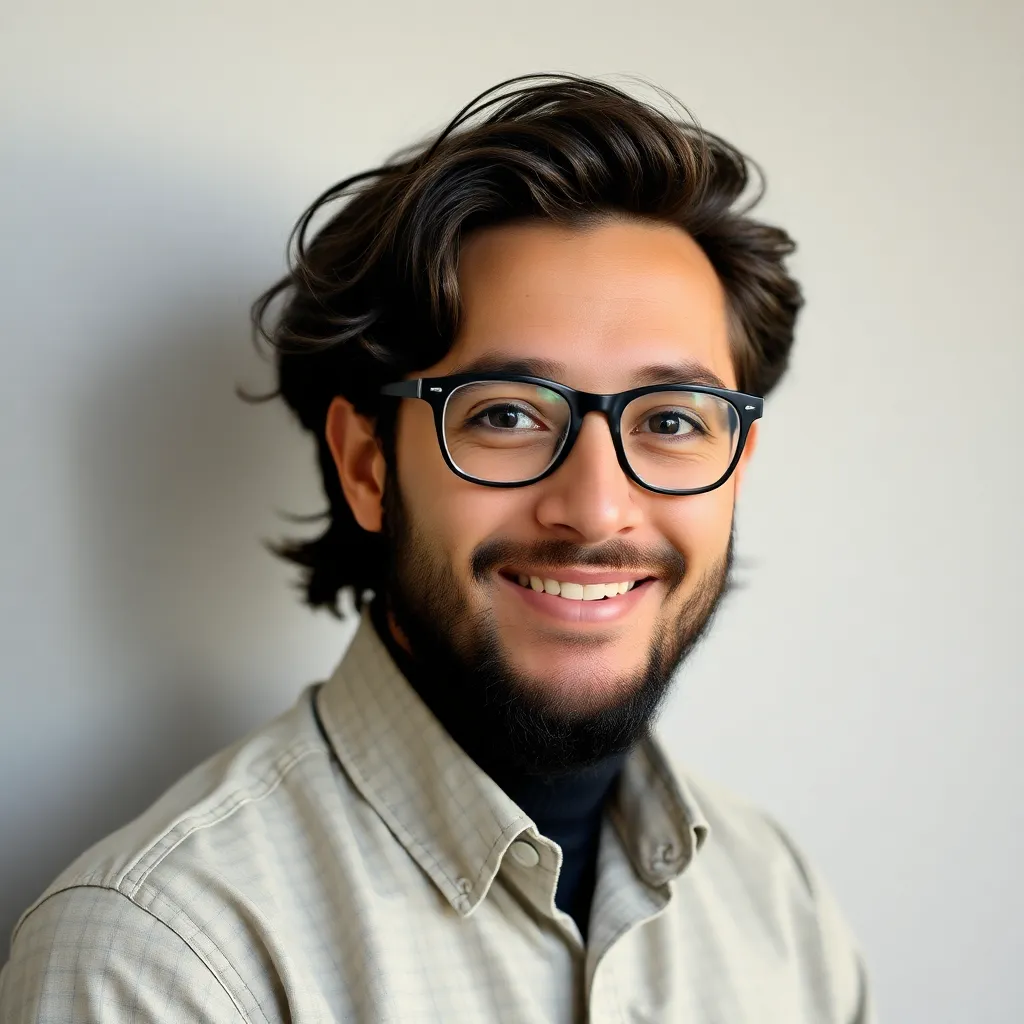
Kalali
Apr 24, 2025 · 8 min read

Table of Contents
What is Found in RNA But Not DNA? A Deep Dive into Ribonucleic Acid's Unique Composition
RNA, or ribonucleic acid, plays a crucial role in translating the genetic information stored in DNA into functional proteins. While both RNA and DNA are nucleic acids built from nucleotides, they possess several key differences in their structure and function. Understanding these distinctions is critical to comprehending the complexities of cellular processes and genetic expression. This article delves into the unique components and modifications found in RNA but not in DNA, highlighting their significance in various biological pathways.
Meta Description: Explore the key structural and functional differences between RNA and DNA. Discover the unique components and modifications found exclusively in RNA, including its ribose sugar, uracil base, and diverse secondary structures. Learn about the implications of these differences for RNA's multifaceted roles in gene expression and regulation.
The most fundamental difference lies in their sugar-phosphate backbone. DNA employs deoxyribose, a sugar lacking a hydroxyl (-OH) group on the 2' carbon, while RNA utilizes ribose, which contains a hydroxyl group at this position. This seemingly minor difference has significant implications for the molecules' stability and three-dimensional structure. The presence of the 2'-hydroxyl group in RNA makes it more susceptible to hydrolysis, rendering RNA less stable than DNA. This inherent instability is, however, crucial for RNA's transient roles in gene expression. DNA, on the other hand, needs to be highly stable to maintain the integrity of the genetic code across generations.
1. The Ribose Sugar: A Defining Characteristic
As mentioned, the presence of the 2'-hydroxyl group on the ribose sugar of RNA is a defining characteristic absent in DNA's deoxyribose. This hydroxyl group contributes to RNA's increased reactivity and susceptibility to degradation. The additional hydroxyl group also influences the conformation of the RNA molecule, impacting its secondary and tertiary structures. The increased flexibility conferred by the 2'-OH group allows RNA to adopt a wider variety of complex three-dimensional shapes, vital for its diverse functional roles. These complex structures are often critical for RNA's interactions with proteins and other nucleic acids.
The 2'-hydroxyl group's influence extends beyond simple structural differences. It actively participates in RNA catalysis, a key function of some RNA molecules (ribozymes). The hydroxyl group acts as a nucleophile, participating in the cleavage and formation of phosphodiester bonds. This catalytic activity is crucial for processes like RNA splicing and ribosome function. DNA, due to its lack of the 2'-OH group, lacks this catalytic potential.
2. Uracil vs. Thymine: A Base Pair Substitution
Another critical distinction lies in the nitrogenous bases. While both DNA and RNA utilize adenine (A), guanine (G), and cytosine (C), they differ in the fourth base. DNA employs thymine (T), while RNA uses uracil (U). Both uracil and thymine are pyrimidines, but they differ by a single methyl group. Thymine possesses a methyl group at the 5 position, while uracil lacks this modification.
The absence of the methyl group in uracil makes it more susceptible to spontaneous deamination, converting it to cytosine. This deamination can lead to mutations if not corrected by cellular repair mechanisms. The methyl group in thymine offers protection against this spontaneous deamination, thus enhancing the stability of the DNA molecule. The evolutionary selection for thymine in DNA likely reflects this stability advantage. The use of uracil in RNA, despite its susceptibility to deamination, might be linked to its transient nature and the higher turnover rates associated with RNA molecules. The cost of greater instability is apparently outweighed by other functional advantages.
3. The Diverse World of RNA Modifications: Beyond the Canonical Bases
Beyond the fundamental differences in sugar and base composition, RNA showcases a remarkable diversity of post-transcriptional modifications. These modifications alter the structure and function of RNA molecules, expanding their versatility considerably. These modifications are largely absent in DNA.
-
Methylation: Methylation of RNA bases, such as N6-methyladenosine (m6A), 5-methylcytosine (m5C), and pseudouridine (Ψ), is common and influences RNA stability, splicing, translation, and other cellular processes. These modifications are highly dynamic and regulated, contributing to the complexity of gene expression.
-
Pseudouridine (Ψ): This isomer of uridine is found extensively in tRNA and rRNA, affecting their structure and function. Pseudouridine enhances base-stacking interactions, stabilizing RNA secondary structures. It can also alter codon recognition and influence translation efficiency.
-
Inosine (I): Inosine, a deaminated form of adenosine, is found in tRNAs and contributes to wobble base pairing. This wobble base pairing allows a single tRNA to recognize multiple codons, enhancing the efficiency of translation.
-
Ribose Modifications: Modifications to the ribose sugar itself also occur, influencing RNA folding and stability. These can involve methylation or other chemical alterations to the ribose moiety.
These and other modifications highlight the dynamic nature of RNA molecules and their regulatory potential. The specific modifications found in an RNA molecule can be highly informative, revealing its functional context and role within the cell. Such intricate regulatory mechanisms are largely absent in DNA, where the primary focus is on maintaining the integrity of the genetic code.
4. Secondary and Tertiary Structures: RNA's Functional Fold
While DNA typically exists as a double helix, RNA displays a far greater diversity of secondary and tertiary structures. These complex three-dimensional arrangements are crucial for RNA's diverse functional roles. The single-stranded nature of RNA, coupled with the flexibility conferred by the 2'-hydroxyl group, allows it to form intricate folds, including hairpin loops, internal loops, bulges, and multi-branched junctions.
These structures are often critical for RNA's interactions with proteins and other molecules. For example, the specific tertiary structure of tRNA is essential for its function in protein synthesis. The cloverleaf structure of tRNA ensures the correct positioning of the anticodon loop for codon recognition and amino acid delivery. Similarly, the complex tertiary structure of ribosomal RNA (rRNA) is essential for the ribosome's catalytic activity in peptide bond formation. DNA's double helical structure, while crucial for its function, is comparatively less complex and structurally diverse.
5. RNA's Catalytic Potential: Ribozymes
Some RNA molecules possess catalytic activity, acting as enzymes called ribozymes. This catalytic capability is directly linked to the presence of the 2'-hydroxyl group on the ribose sugar. Ribozymes are involved in various crucial cellular processes, including RNA splicing, RNA cleavage, and peptide bond formation. The most prominent example is the ribosome itself, a ribonucleoprotein complex where rRNA plays a catalytic role in protein synthesis.
The catalytic activity of ribozymes challenges the central dogma of molecular biology, which initially posited that only proteins could act as enzymes. The discovery of ribozymes underscored RNA's crucial role in the early evolution of life, suggesting that RNA might have served as both a carrier of genetic information and a catalyst in prebiotic environments. DNA, lacking the 2'-hydroxyl group and its inherent reactivity, lacks this catalytic potential.
6. Functional Diversity: Beyond Messenger RNA
While DNA primarily serves as a repository for genetic information, RNA exhibits a far greater functional diversity. While messenger RNA (mRNA) carries genetic information from DNA to the ribosome, several other types of RNA play essential roles in gene expression and regulation:
-
Transfer RNA (tRNA): tRNAs deliver amino acids to the ribosome during protein synthesis. Their unique cloverleaf structure is crucial for their function.
-
Ribosomal RNA (rRNA): rRNAs form the structural and catalytic core of the ribosome. They participate directly in peptide bond formation.
-
Small nuclear RNA (snRNA): snRNAs are involved in RNA splicing, a process that removes introns from pre-mRNA.
-
Small nucleolar RNA (snoRNA): snoRNAs guide the modification of other RNAs, such as rRNA and tRNA.
-
MicroRNA (miRNA): miRNAs regulate gene expression by binding to target mRNAs and inhibiting their translation or promoting their degradation.
-
Small interfering RNA (siRNA): siRNAs are involved in RNA interference (RNAi), a mechanism for silencing specific genes.
-
Long non-coding RNA (lncRNA): lncRNAs are a diverse group of non-coding RNAs with various regulatory functions. Their roles are still under active investigation, but they are known to be involved in gene regulation, chromatin remodeling, and other cellular processes.
This diversity of RNA types and functions highlights the multifaceted roles of RNA in cellular processes. DNA, in contrast, maintains a more focused role as a stable repository of genetic information.
7. RNA's Role in Gene Regulation: A Dynamic Player
RNA plays a significant role in regulating gene expression at multiple levels. This regulatory capacity distinguishes it from DNA, whose primary function is to store genetic information. RNA-based regulatory mechanisms include:
-
Transcriptional Regulation: Some non-coding RNAs interact with DNA and proteins to modulate gene transcription.
-
Post-transcriptional Regulation: miRNAs and siRNAs regulate gene expression by affecting mRNA stability and translation.
-
RNA Editing: RNA editing processes modify the nucleotide sequence of RNA molecules, altering their function.
-
RNA Splicing: Alternative splicing of pre-mRNA generates multiple protein isoforms from a single gene, expanding the proteome’s diversity and regulatory capacity.
This intricate network of RNA-based regulatory mechanisms underscores the dynamic nature of gene expression and its responsiveness to environmental cues. DNA, while involved in setting the stage for gene expression through its sequence, plays a less direct and dynamic role in the fine-tuning and regulation of gene expression compared to RNA.
Conclusion: RNA’s Unique Attributes and Biological Significance
In summary, RNA and DNA, while both nucleic acids, differ significantly in their composition, structure, and function. The presence of the 2'-hydroxyl group on ribose, the use of uracil instead of thymine, and the remarkable diversity of RNA modifications all contribute to RNA's unique properties. RNA's inherent instability, its ability to fold into diverse three-dimensional structures, and its catalytic potential distinguish it from DNA, allowing it to perform a wide range of vital biological functions beyond simply storing genetic information. Understanding these unique attributes of RNA is essential for comprehending the complexity of cellular processes and the intricacies of gene regulation. Ongoing research continues to reveal the surprising depth and breadth of RNA’s roles in life, highlighting its significance as a dynamic and versatile molecule.
Latest Posts
Latest Posts
-
4 Out Of 18 As A Percentage
Apr 24, 2025
-
How Many Groups Of Are In
Apr 24, 2025
-
11 5 Inches Is How Many Cm
Apr 24, 2025
-
127 Cm Is How Many Inches
Apr 24, 2025
-
What Percent Of 192 Is 48
Apr 24, 2025
Related Post
Thank you for visiting our website which covers about What Is Found In Rna But Not Dna . We hope the information provided has been useful to you. Feel free to contact us if you have any questions or need further assistance. See you next time and don't miss to bookmark.